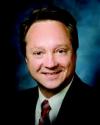
Fall 2013 - Vol. 8, No. 3
The Perfect Storm: Worsening Microbial Resistance and Too Few New Antibiotics?
Joseph Kontra, M.D.
Infection Specialists of Lancaster
ABSTRACT
The appearance of highly virulent and broadly resistant microbes such as Carbapenem Resistant Enterobacteriaecea (CRE) has lead some to predict the onset of the Post-antibiotic Era, in which infections are untreatable by any currently available antibiotics. This paper examines the ancient origin of antimicrobial resistance and its implications. It also explores the reasons for the dearth of new antibiotics and the measures being taken to reverse that draconian trend. As it turns out, the wake up call may not be too late.
“More wisdom is latent in things-as-they-are than in all the words men use”
Antoine de Saint-Exupèry
THE NATURE OF ANTIBIOTICS AND BACTERIAL RESISTANCE
Antibiotics are naturally occurring chemical compounds produced by a variety of organisms, predominately fungi, which function in nature to suppress bacteria and thus preserve an ecologic niche. Antibiotics are, and always have been, an integral part of the natural ecology of our planet.
Recall that the discovery of penicillin, one of the greatest events in the history of Medicine, resulted from Fleming’s astute observation that a mold contaminant on a Petri plate inhibited the growth of the surrounding bacteria. In fact, even today about 80% of currently available antibiotics are derived either directly or indirectly from fungi. As therapeutic antibiotics have only been in use for about 70 years, one might suspect that resistance to antibiotics would also be a relatively modern phenomenon, and that pre-antibiotic microbes from prior to 70 years ago should therefore be highly susceptible to antibiotic agents. This narrow anthropomorphic view, however, is far from the truth.
Bacteria and fungi have existed on this planet for more than 40 million years, i.e. prior to the appearance of humans, let alone the birth of Fleming. The natural occurrence of ancient antibiotic agents as primary weapons in the ecologic wars of the early earth‘s soil, water, and vegetation was accompanied by the evolution of countless bacterial resistance mechanisms equally as ancient.
A Canadian study of 30,000 year-old bacterial genes resurrected from permafrost sediments in the Yukon has shifted the foundations of our understanding of microbial resistance.1 Utilizing DNA analysis techniques from forensics and archeology, extraction of Pleistocene era bacterial DNA from soil Actinobacteria demonstrated genes that produce resistance to modern antibiotics such as penicillin, tetracycline, and even vancomycin. The later gene, from the age of the wooly mammoth, demonstrated remarkable similarities of sequence and function to the modern vancomycin resistance gene thought to have originated in the 1980’s.
Lechuguilla Cave in Carlsbad Cavern National Park in New Mexico has been sealed off from any contact with surface air or water for an estimated 4-7 million years, yet from this ancient pristine site 400 meters below the surface, researchers were able to cultivate 93 strains of bacteria.2 Most strains harbored resistance genes to one or more of the 26 antibiotics tested, and some demonstrated resistance to as many as 14 antibiotics, including ampicillin, telithromycin, and even the recently developed daptomycin. Within these complex soil microbiomes there may exist many as yet undiscovered antibiotics, which microbes millennia ago conquered.
Given the weight of evolutionary ‘experience’ on the side of bacteria, is it any wonder that our every attempt at new antimicrobial development is summarily dismissed with the rapid development of resistance by the target microbe? Are we, like Sisyphus, doomed to endlessly repeat the same ultimately hopeless task?
Not necessarily, and nature can show us the way. To that point consider the recent report of a newly discovered antibiotic anthracimycin.3 This compound, discovered by scientists at the Scripps Institute of Oceanography, is a natural product of ocean sediment actinomycetes. It has a unique mechanism of action, with potent antibacterial activity including against both anthrax and MRSA. This, along with similar discoveries derived from ancient microbes, could potentially spawn whole new classes of therapeutic antibiotics for human use.
THE CURRENT MAJOR THREATS
Prior to the new millennium, the great worry among Infectious Diseases clinicians was the increasing resistance among Gram-positive organisms (GPO). The problem of infections with Methicillin-Resistant Staph Aureus (MRSA) in hospitals and nursing homes, which began in the 1970’s, continued to slowly spread. In addition, the arrival of community acquired MRSA, yet another new and more virulent step in microbial evolution, exploded on the scene in the early 2000’s. And while Vancomycin-Resistant Enterococcus Faecium (VREF) remained a persistent low-level threat, the great fear was the possible spread of the resistance gene for Vancomycin from VREF to MRSA, resulting in the “superbug” Vancomycin-Resistant Staph Aureus (VRSA). All of this led to the urgent development and release of several new antibiotics aimed at these multi-drug resistant (MDR) GPO. Fortunately, the global threat of VRSA has never materialized, and an adequate supply of effective antibiotics for MDR GPO is now part of the Infectious Diseases armamentarium.
Epidemiologic research has in fact identified a cadre of resistant bacteria to be the focus of study due to their multi-drug resistant nature and propensity to wreak havoc in hospitals and nursing homes. These are the so-called ESKAPE pathogens (Entercoccus faecium, Staphylococcus aureus, Klebsiella pneumoniae, Acinitobacter baumannii, Pseudomonas aeruginosa, and Enterobacter). Note that several Gram-negative bacilli (GNB) have now been added to the list of multi-drug resistant “superbugs.”
It is MDR GNB that have in fact now become perhaps the greatest threat to patients. Common GI organisms such as E. coli and Klebsiella have acquired a series of Extended-Spectrum Beta- Lactamase enzymes (ESBL), rendering them resistant to all penicillins and cephalosporins. These pathogens are treatable only with carbapenem antibiotics, considered the last line of defense in terms of available active antibiotics against GNB. These organisms have become prominent pathogens in nursing homes and hospitals, with high rates of morbidity and mortality, and they have demonstrated a propensity for nosocomial spread. In addition, as the ESBL GNB are GI microbes, development of a chronic stool-carrier state led both to a tendency to recurrent infections in patients, and a difficult challenge for eradication.
All too soon to follow was the development of Carbapenem-Resistant Enterobacteriaceae (CRE). These deadly pathogens have spread worldwide in less than a decade, now posing an increasing mortality threat especially to debilitated patients, and to patients in intensive care units. The resistance genes that carry these enzymes are readily transferred between organisms on extra-chromosomal plasmids, making their spread among Klebsiella strains within the orgy of gastrointestinal microflora all too easy. The most infamous example of this is the New Delhi Metallo-Beta-Lactamase (NDM-1). This enzyme, first identified in India in 2009, spread quickly in GNB around the world in only a few months, leaving a swath of devastating infections in its wake. Fortunately, the prevalence of NDM-1 in the United States has so far remained low. In the United States the scene is dominated by the Klebsiella pneumoniae carbapenemase (KPC) enzyme, with equally morbid consequences, as exemplified by the well-publicized 2011 outbreak of CRE at the Clinical Center of the National Institutes of Health (NIH).
According to the CDC,4 the incidence of CRE increased from less than 1% in 2000 to 8% in 2007. In Europe, epidemiologic surveillance indicated that in 2010 rates of CRE were as high as 16% in Italy, and 59% in Greece. These organism cause a wide variety of clinical infections including catheter related bacteremias, hospital associated pneumonia, surgical site and wound infections, UTIs, and endocarditis. Infections are preceded by colonization of the gastrointestinal tract. Mortality rates in ICUs are reported to be as high as 60%, and nosocomial outbreaks around the world have proved devastating.
Treatment of CRE is critically problematic. Since CRE are by nature resistant to the last line of defense in the form of carbapenem antibiotics, only a few relatively ineffective drugs remain, including tigecycline, and the resurrected old drug colistin. Both suffer from limited efficacy and significant toxicities. Combination therapy has been tried, including triple therapy that includes the very carbapenems to which the organisms are resistant, with unfortunately very limited success. Emphasis has thus turned to strict enforcement of heightened infection control protocols to attempt to limit the spread of these deadly pathogens within the hospital.
The great fear of course, and one which in some centers has come to fruition, is that these CRE pathogens will become resistant to the relatively weak drugs listed above. The result is infection with a hyper-virulent, deadly, rapidly spreading pathogen for which we have no antibiotics: a Doomsday Microbe that would herald the Post-antibiotic era where deadly infections are, as they were in the 1920’s, simply untreatable.5 The failure of modern medicine to keep pace with advancing microbial resistance may cause us not only to fall into the abyss, but to be so late in our response as to have passed our Rubicon as well.
RESPONSE TO THE WAKE UP CALL
Antibiotic research and development has traditionally not been attractive to drug manufacturers, who much prefer the higher profits from drugs that need to be prescribed long-term to control diseases rather than to cure them. Since 2002 the Infectious Diseases Society of America (IDSA) has warned of the consequences of the dwindling pace of new antibiotic development (see Table 1) that had been occurring at the same time as the escalation in the number and variability of MDRO, especially the rise of the MDR GNB. In their 2009 report, in fact, the IDSA noted that despite earlier calls to action, no new antibiotics with a potent GNB spectrum had reached even phase II trials. The profit motive of the drug manufacturers and the tedious and protracted approval process of the US Food and Drug Administration (FDA) remained barriers that seemed insurmountable. The drug pipeline was obstructed, and its draconian implications were frightening.
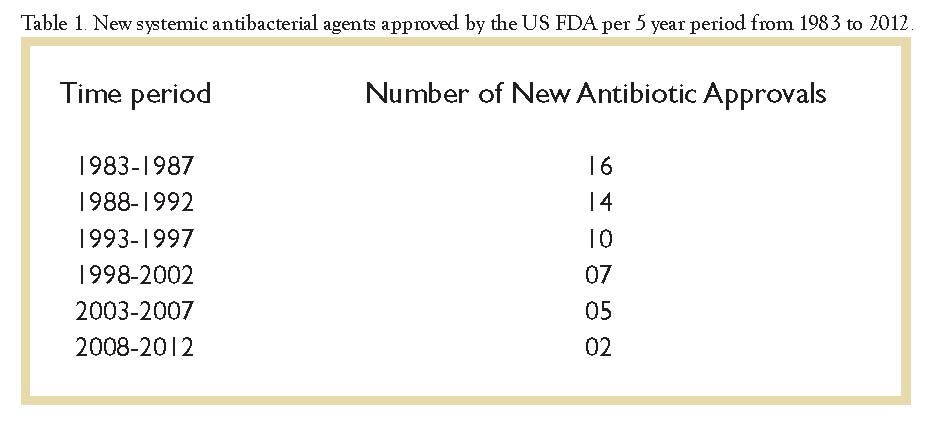
Subsequently in 2010, the IDSA testified before Congress and later announced its “10 x ‘20 initiative” calling for a global commitment to develop and release 10 new potent antibiotics active against MDRO by the year 2020.6 The Society has worked vigorously with the FDA, the US National Institutes of Health, the Centers for Disease Control and Prevention, and the World Health Organization. Over 20 major organizations representing medical and professional societies, public health agencies, patient advocacy groups, health systems, and other stakeholders have supported economic and legislative changes designed to help reach the 10 x ‘20 goal.
It seemed clear that to reach this goal, fundamental changes in the process of drug development must occur. The IDSA has proposed, and the FDA is being urged my members of Congress to adopt, a fast track process for research and development of new antimicrobial agents. Drugs developed in this fashion would be granted a special FDA label specifying that their use is limited to specific patient populations with specific well-documented high-risk infections. In addition, consideration for extending drug patents for an additional 5 years has been proposed to help incentivize development of new drugs. While there have been objections and cautions raised about such a mechanism, bipartisan support is growing.
Financial incentives for drug manufacturers are also an integral part of the overall strategy.7 The Health and Human Services Department recently announced that it will pay $40M to GlaxoSmithKline, with a potential of $200M over 5 years, to develop antibiotics to combat MDRO and bioterrorism agents. And in a unique act of corporate cooperation, GlaxoSmithKline and AstraZeneca recently became part of a joint government and industry effort to develop the needed antibiotic agents effective against GNB MDRO, a collaborative effort which has uncharacteristically included pooling of research data and resources.
The overwhelming tonnage of antimicrobials used in food production, however, continues to dwarf that of use in medical therapeutics, and thus contributes mightily to the problem of antimicrobial resistance. Legislation has been proposed to limit the overuse of antibiotics in animals in the food industry. While this effort faces an uphill battle in Congress, there is no doubt that this problem will also need to be effectively addressed if we are to be ultimately successful in turning the tide of microbial resistance.
The synergy of governmental and industry efforts has already begun to bear fruit. Several new antibiotic agents with excellent activity against MDR GNB, particularly CRE, are now in phase II and III trials. Several of these are combination agents that utilize the newly developed beta-lactamase inhibitor avibactam (AB). This compound differs from the existing beta-lactamase inhibitors (clavulanic acid, sulbactam, and tazobactam) in that it does not contain a beta lactam core structure, thus rendering it uniquely resistant to carbapenemases and extended spectrum beta lactamases. These combination agents include ceftazidime-AB, aztreonam-AB, ceftaroline-AB, and imipenem-AB. A total of seven promising agents have been identified in a recent IDSA update.8
While there is still much ground to cover, the release of these agents over the next few years may signal a turning of the tide in our battle against MDRO, and the beginning of the long climb out of the abyss.
REFERENCES
1. D’Costa VM, et. Al. Antimicrobial Resistance is Ancient Nature. 2011 DOI: 10.1038/nature10388
2. PLoS ONE April 11,2012
3. Jang, Hwa Jang; Nam, SJ, Locke, JB, et al. Anthracimycin, a potent anthrax antibiotic from a marine-derived actinomycete. Angewandte Chemie International Edition 52 (30): 7822–7824
4. MMWR Vital Signs: Carbapenem-Resistant Enterobacteriaceae: 62(09);165-70
5. Levermore, DM. Has the era of untreatable infections arrived? J. Antimicrob. Chemother. 64, i29-i36 (2009)
6. Infectious Diseases Society of America. The 10 x ‘20 initiative: pursuing a global commitment to develop 10 new antibacterial drugs by 2020. Clin Infect Dis 2010; 50:1081-3
7. www.nytimes.com/2013/06/03/health/experts-debate-plan-to -speed-antibiotic-development
8. Boucher, HW. 10 x ’20 Progress–Development of New Drugs Active Against Gram-Negative Bacilli: An Update From the Infectious Diseases Society of America. Clin Infect Dis 2013;56(12):1685-94.