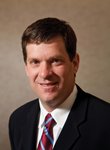
Summer 2013 - Vol. 8, No. 2
The Cyberknife® M6TM Radiosurgery System
Jeffrey S. Eshleman, M.D.
Kenneth G. Berkenstock, M.D.
Kishur P. Singapuri, M.D.
Lancaster Radiology Associates, Inc.
Charles Fuller, Ph.D.
Lancaster General Hospital
“Innovation distinguishes between a leader and a follower.”
— Apple co-founder Steve Jobs
ABSTRACT
Lancaster General Health is the first institution in the United States to have the next generation CyberKnife made by Accuray Inc., the M6 series (Fig. 1). The CyberKnife utilizes non-invasive stereotactic radiosurgery to deliver precise, high doses of radiation to targets anywhere in the body. Recent studies have shown that stereotactic radiosurgery offers high rates of tumor control with minimal toxicity, which has led to significant growth in its use as an effective alternative to conventional surgery for many small tumors and selected medical conditions. The new M6 series CyberKnife is the most advanced robotic system available today and with its new InCise multileaf collimation will expand indications and the number of patients who may be eligible for CyberKnife treatment.
Fig. 1: CyberKnife M6 Series Robotic Radiosurgery System
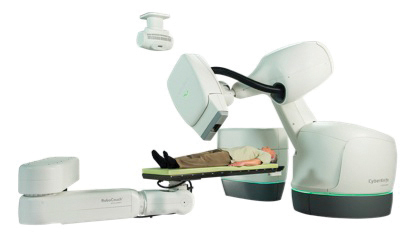
INTRODUCTION
Though its name may suggest scalpels and surgery, CyberKnife treatment is a non-invasive method of treating tumors anywhere in the body with precise, high doses of radiation. It offers a non-surgical option for many patients who have inoperable or surgically complex tumors, or who may be seeking an alternative to surgery.
Over the past decade, the field of radiation oncology has undergone significant changes. Driven by advances in technology, our ability to better image, localize, and track a tumor has grown exponentially. Radiation oncologists are now more confident than ever that their target is encompassed by the treatment while adjacent normal tissues and organs are maximally spared the effects of radiation. As confidence has grown, shorter treatment regimens with much higher doses of radiation have emerged, allowing greater control of tumors, fewer side effects, and increased patient convenience. And most recently, there has been a significant increase in the utilization of very high, “ablative” doses of radiation to treat tumors in one or a few fractions, methods that are called stereotactic radiosurgery (SRS) and stereotactic body radiotherapy (SBRT), respectively.
STEREOTACTIC RADIOSURGERY (SRS)/STEREOTACTIC BODY RADIOTHERAPY (SBRT)
The word stereotactic is derived from the Greek word στερεoς (stereo), which translates to “solid” and the Greek word τακτική (taxis), a Greek military term, meaning “arrangement or order” or “tactic.” The modern medical definition of stereotactic is the utilization of a surgical technique for precisely directing an instrument or beam of radiation in three planes using ordinance systems provided by medical imaging to reach a specific locus in the body. Today, the delivery of radiation is considered stereotactic only if it uses advanced imaging techniques and state-of-the-art treatment and image guidance technologies to precisely deliver radiation to the intended target in either a single (SRS) or a few (up to 5) treatments (SBRT). SRS and SBRT are used in the treatment of many types of benign and malignant tumors, as well as other pathologies (Table 1). However, in theory, any organ or target within the body can be targeted with stereotactic radiosurgery.
Unlike SRS and SBRT, conventional radiotherapy is not ablative, and is given in daily doses that are smaller and sublethal, are fractionated over multiple weeks, and often include a larger volume of irradiated tissue. Normal organs are often included within the radiation target volume due to limitations of radiation delivery techniques or non-discrete target volumes. However, multiple low-dose radiation treatments do allow normal cells to repair sublethal damage between treatments and increases their chance of survival, whereas many tumor cells have mutations in DNA repair pathways that make them less likely to survive even sub-lethal doses of radiotherapy.1 Thus, fractionated radiotherapy creates a therapeutic window in which the same overall radiation dose kills more tumor cells than normal cells. Unfortunately, this therapeutic window is often small, and to control tumors, higher radiation doses are required than surrounding normal tissues can tolerate. Thus, often either the tumor cannot be locally controlled (too low a dose) or surrounding normal tissues are damaged beyond repair (too high a dose).
The rapid technological advances in radiation oncology and imaging have mitigated these problems. Higher and higher doses of radiation can be delivered to targets more precisely, increasing the chances that tumors can be controlled without exceeding the limits of radiation that can be tolerated by surrounding normal organs. These advances have led to a significant widening of the therapeutic window in fractionated radiotherapy, and clinical data reveal significant improvements in outcomes. An example is the routine use of intensity-modulated radiation therapy with image guidance for prostate cancer. By increasing the therapeutic ratio with advanced technology, higher doses of radiation have led to higher cure rates and less immediate and long-term toxicity.
Because target volumes are now well defined, and can be localized and tracked by state-of-the-art radiotherapy systems, very high ablative doses of radiation therapy (SRS and SBRT) can be delivered, making it extremely important to have the most sophisticated radiosurgery system because unlike the case with conventional fractionated radiotherapy, all tissues within the target volume of ablative radiation treatment are now at risk for damage beyond repair. Still, because of more precise localization and tracking, the therapeutic window is increased, and the target can be ablated while high dose radiation to the surrounding normal tissues is minimized or avoided.
BRIEF HISTORY OF STEREOTACTIC RADIOSURGERY
Stereotactic radiosurgery was first developed to treat intracranial lesions non-invasively. In 1951, the Swedish neurosurgeon Dr. Lars Leksell developed the concept using many radiation beams that were focused at a target with a three-dimensional coordinate system and rigid skull fixation by means of a metal frame fixed to the skull with screws.2 This technique produced highly conformal high dose radiation distributions while controlling for patient movement to sub-millimeter precision. His concepts led to the development of the Gamma Knife radiosurgery system in 1972, using the aforementioned frame fixed to the skull for exact sterotaxy, and intracranial targets, typically defined by CT or MR imaging, that are localized utilizing a three-dimensional coordinate system. Two hundred and one fixed radioactive cobalt sources are focused to a fixed isocenter to treat intracranial tumors with great precision and accuracy.3,4
The Gamma Knife unit is still one of the leading stereotactic systems in the world, but its use is limited to treating intracranial pathologies and it requires placement of an invasive frame to ensure that there is minimal effect from patient movement during treatment. Due to the need for an invasive skull frame, treatment was limited to one episode, thus eliminating the advantage of fractionating treatment (repair of sublethal damage and improved therapeutic window) and reducing the number of patients potentially eligible for treatment. In the mid-1980’s, conventional linear accelerators—the main radiotherapy delivery systems for radiation oncology departments—were retrofitted to allow for SRS treatment. Since these conventional linear accelerators (linacs ) were commonplace, use of intracranial SRS was able to expand greatly. However, early linac-based SRS systems often had deficient technique, poor or absent image guidance, and limited treatment configurations. Like the Gamma Knife, linac-based SRS was mainly limited to intracranial targets. For extra-cranial sites, it could not reliably address the problems of patient movement or internal motion.
THE CYBERKNIFE SYSTEM
The CyberKnife system was invented by John R. Adler, a Stanford University Professor of Neurosurgery and Radiation Oncology, and Peter and Russell Schonberg of Schonberg Research Corporation. After completing a fellowship in Sweden with Lars Leksell, MD, Adler had a vision of creating a non-invasive robotic radiosurgery system with superior accuracy for treatment of tumors anywhere in the body.5,6
Unlike most linac-based systems, the CyberKnife was designed specifically to deliver stereotactic radiosurgery, and it overcame the main limitations of conventional linear accelerators by allowing 1) the ability to continually track, detect, and correct for tumor and patient movement, and 2) a significant expansion of treatment configurations utilizing so-called intelligent robotics. Real-time target tracking and correction not only eliminated the need for an invasive fixation device for intracranial targets, but it made the benefits of radiosurgery available for tumors elsewhere.
THE CYBERKNIFE TECHNOLOGY
CyberKnife is manufactured by Accuray® headquartered in Sunnyvale, California. The first patient was treated on CyberKnife in 1994, and a prototype unit was used between 1994 and 2001. CyberKnife received FDA approval in 2001, and the first FDA-approved CyberKnife was installed at Stanford University in October 2001. The first installation of the CyberKnife M6 series in the United States was at Lancaster General Hospital in May 2013. The two main elements of the CyberKnife treatment system are (1) radiation produced from a small linear particle accelerator is on a robotic arm, which allows megavoltage x-rays to be directed at any part of the body from nearly any direction; and (2) real-time images of the target are tracked with a frameless system. These two essential features set CyberKnife apart from other stereotactic modalities.
1. Robotic-mounted linear accelerator
The radiation of CyberKnife comes from a robotic arm mounted on a general-purpose industrial robot (German KUKA KR 240), the same robotic technology used to manufacture BMW automobiles. This robotic mounting allows near-complete freedom to direct radiation to the target from nearly any direction, in effect providing over 1,200 radiation shooting angles. The robotic mounting allows very fast repositioning without the need to move the patient, unlike current conventional radiotherapy with linac-based gantry configurations. In addition, robotic technology allows for the real-time correction of patient and target motion.
2. Real-time image guidance with target tracking allows for a frameless delivery system
The CyberKnife continually detects, tracks, and corrects for tumor motion throughout treatment, and there are several options to accomplish these objectives: a) taking x-rays periodically during treatment of bony anatomy; b) using metal markers placed within the body; and c) as exemplified by lung cancer, using differences in soft tissue densities. Tracking is accomplished by light-emitting optical fibers mounted on the patient and tracked using a CCD (Charge Coupled Device) camera. The tracking system is utilized primarily for lung, liver, pancreatic and other tumors that move significantly because of respiratory motion.
ADVANTAGES OF A FRAMELESS SYSTEM
Aside from the obvious advantages for patient comfort, a frameless stereotactic system allows either single or multi-session treatment. As some tumors abut or overlap critical structures, they may be untreatable in one fraction, or would require under-dosing of the tumor or over-dosing of critical structures. By dividing the radiation dose into even a few fractions, healthy tissue may be kept within tolerance levels while not sacrificing control of the tumor. There are now indications for treatment of tumors of the lung, prostate, spine, liver, and pancreas and the field continues to expand rapidly.
THE NEW M6 SERIES
The M6 series will be the first CyberKnife system to have the InCise Multileaf Collimator, a device made up of individual leaves that can move independently in and out of the radiation beam to modulate its intensity and shape. This new technology will greatly expand the selection of patients eligible for CyberKnife treatment to include those with larger or more irregular shapes, while also perhaps decreasing treatment times from an average of 30-45 minutes to approximately 15-20 minutes.
With these improvements, even patients who are currently not eligible for SRS or SBRT (patients requiring highly conformal but not ablative dose of radiotherapy) may benefit from the continual image guidance, tumor tracking with automatic correction, and non-isocentric, non-coplanar treatment delivery with sub-millimeter accuracy that the CyberKnife system provides.
EXAMPLE OF CASES UTILIZING CYBERKNIFE SRS & SBRT
Intracranial Tumors: SRS has been used to treat intracranial lesions with great success for several decades. The most commonly treated entities are brain metastases, meningiomas, acoustic neuromas, gliomas, A-V malformations, as well as functional disorders such as trigeminal neuralgia and movement disorders. Fig. 2 shows a highly conformal SRS plan for a typical patient with a cerebellar brain metastasis treated with CyberKnife technology. For brain metastases, SRS is more than 80% effective in achieving local control.7,8,9
Fig. 2. Three-dimensional and two dimensional images of a CyberKnife treatment plan for a single left cerebellar brain metastasis.
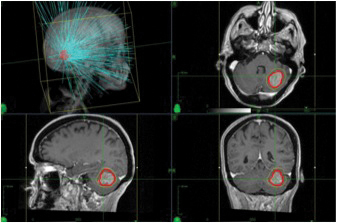
Early Stage Non-small Cell Lung Cancer: In patients with early-stage non-small cell lung cancer, CyberKnife SBRT achieves local control in 85% of patients in studies with over 3 years of follow-up. Patients are typically treated in 3 to 4 fractional doses up to total doses of 48.0 to 60 Gy (the Gray is the unit of absorbed radiation). Significant acute or long-term toxicity is rare and there is excellent preservation of lung function as doses of radiotherapy are limited to the tumor volume plus a small margin as demonstrated in the fig. 3.10,11,12
Fig. 3. Three-dimensional and two dimensional images of a CyberKnife treatment plan for an early stage non-small cell lung carcinoma.
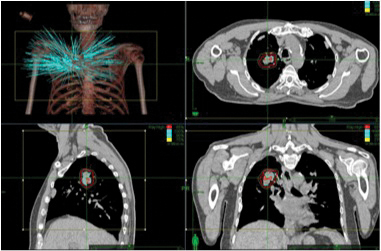
Prostate Cancer: Prostate cancer can be treated with stereotactic techniques. The traditional protocol included daily (Mon.-Fri.) treatments for approximately 9 weeks. With the precision and motion tracking ability of CyberKnife, a full course SBRT prostate treatment can be completed in five fractions. Treatment is highly conformal (Fig. 4). Also, besides being more convenient for patients, treatment is more cost effective compared to traditional 8-9 week radiotherapy.13,14,15
Fig. 4. Three dimensional reconstruction of beam geometries for an intermediate risk prostate cancer patient.
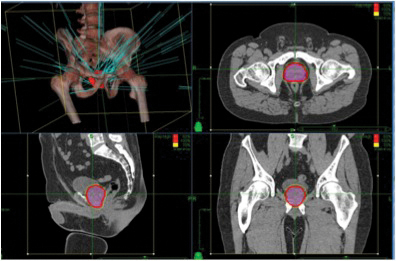
LIMITATIONS, RISKS AND COST OF TECHNOLOGICAL ADVANCES IN RADIATION ONCOLOGY
Advanced radiation technologies such as CyberKnife are not indicated for many malignancies that can be treated with simpler and lower cost techniques with similar outcomes. In addition, some tumors may be better suited for other radiation delivery systems due to differences in image guidance, larger treatment volumes or patient factors such as the ability to lie still for an extended period of time. A comprehensive radiation oncology program will match the appropriate technology to each patient.
As documented in a recent New York Times article, there are rare, but significant, risks associated with the rapid development of new radiation technology.16 To ensure safety, it is essential to have a high quality team including experienced radiation oncologists, medical physicists, dosimetrists, and radiation therapists. This team should provide the highest-level quality assurance program. With very high doses of radiation delivered, the consequences of even a small oversight can lead to significant morbidity or death.
As mentioned previously, the field of radiation oncology is moving toward more precise, higher dose, shorter treatment regimens that will hopefully save lives, provide better quality of life, and due to shorter regimens, save on cost. As the field moves in this direction, it is important to provide treatment locally to allow patients to receive treatment closer to home and family. However, the significant benefits of the recent advances in radiation technology have come at a high cost of equipment, often many millions of dollars. As there is a danger of overutilization to justify the cost, it is imperative that we demonstrate significant clinical benefit of these technologies by moving forward carefully and enrolling patients in clinical trials. Some claim that the parachute effect justifies the cost and implementation of new technology without rigorous research (i.e., you would not require a phase 3 trial to test whether a parachute saves lives). However, many advances in oncology have very little effect on overall survival while greatly increasing the cost of healthcare. If a parachute were less than one percent effective and cost tens of thousands of dollars per jump, would the cost be justified?
CONCLUSION
The discipline of radiation oncology is moving towards shorter, more precise, high dose radiation treatment regimens for many different types of cancer and benign conditions. Accuray’s CyberKnife M6 system is the leader in robotic SRS/SBRT innovation. We are proud to be the first in the United States to offer the new CyberKnife M6 radiosurgery system to our community. As the field of stereotactic radiosurgery and stereotactic body radiosurgery expands, the physicians at the Ann B. Barshinger Cancer Institute will be in the forefront of applying this state-of-the-art technology.
REFERENCES
1. Elkind MM, Sutton-Gilbert H, Moses WB, Kamper C. Sub-lethal and Lethal Radiation Damage. Nature 1967;214: 1088-92.
2. Lunsford LD. Lars Leksell. Notes at the side of a raconteur. Stereotact Funct Neurosurg. 1996–97;67(3–4):153–68.
3. Larsson B, Leksell L, Rexed B, et al. The high energy proton beam as a neurosurgical tool. Nature 1958;182:1222–3.
4. Leksell L. The stereotaxic method and radiosurgery of the brain. Acta Chir Scand 1951; 102:316–19.
5. Adler JR. Stereotactic surgery and radiosurgery. Medical Physics Publication; 1993;237–48.
6. Adler JR, Cox RS. Preliminary experience with CyberKnife – Radiosurgery. Basel: S.Karger; 1996;112–38.
7. Flickinger, J.C. et al. A multi-institutional experience with stereotactic radiosurgery for solitary brain metastases. Int. J. Rad Onc Bio Phys 1994;28:797-802.
8. Sneed, P.K. et al. Radiosurgery for brain metastases: is whole brain radiotherapy necessary? Int J Radiat Oncol Biol Phys 1999;43(3):549-58.
9. Kihlstrom, L. et al. Gamma knife surgery for cerebral metastasis. Acta Neurochir Suppl 1991;52:87-89.
10. Freeman DE, King CR. Stereotactic body radiotherapy for low-risk prostate cancer: five-year outcomes. Radiat Oncol 2011;6:3.
11. King CR, Brooks JD, Gill H, et al. Long-Term Outcomes from a Prospective Trial of Stereotactic Body Radiotherapy for Low-Risk Prostate Cancer. Int J Radiat Oncol Biol Phys 2012;82:887-882.
12. Friedland JL, Freeman DE, Masterson-McGary ME, et al. Stereotactic body radiotherapy: an emerging treatment approach for localized prostate cancer. Technol Cancer Res Treat 2009;8:387-392.
13. Freeman DE, King CR. Stereotactic body radiotherapy for low-risk prostate cancer: five-year outcomes. Radiat Oncol 2011;6:3.
14. King CR, Brooks JD, Gill H, et al. Long-Term Outcomes from a Prospective Trial of Stereotactic Body Radiotherapy for Low-Risk Prostate Cancer. Int J Radiat Oncol Biol Phys 2012;82:887-882.
15. Friedland JL, Freeman DE, Masterson-McGary ME, et al. Stereotactic body radiotherapy: an emerging treatment approach for localized prostate cancer. Technol Cancer Res Treat 2009;8:387-392.
16. Bogdanich, W. ““Radiation Offers New Cures, and Ways to Do Harm”.” New York Times 24 Jan. 2010: 1. Print.