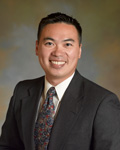
Spring 2012 - Vol.7 - No.1
Radiation Exposure in Trauma Patients
Daniel Wu, D.O.
Associate Medical Director, Trauma Program
Trauma Intensivist, Acute Care Surgeon
INTRODUCTION
Computed tomography (CT) is widely considered one of the most important advances in medicine, especially in the realm of trauma. Many decision algorithms for non-operative management of solid organ injury pivot on access to CT imagery, which is particularly fundamental in the diagnosis and treatment of traumatic brain injury. CT scans are advocated in the course on Advanced Trauma Life Support of The American College of Surgeons – Committee on Trauma.1 But though the diagnostic value of CT scans is not in question, the dramatic increase in their use - from 3 million studies in 1980 to nearly 70 million in 2007 - raises inevitable questions about the side effects of patients’ exposure to so much ionizing radiation.2,3
Compared with conventional radiography, computed tomography exposes patients to much higher radiation doses. Whereas a routine two-view radiograph of the chest typically exposes a patient to approximately 0.1 milliSieverts (mSv), a CT of the chest exposes a patient to more than 100 times that dose of radiation.4 Concerns about the effects of increased exposure to ionizing radiation stems from evidence that links such exposure to the development of cancer. The report on Biological Effects of Ionizing Radiation (BEIR) VII Phase 2 recounts the results of a review conducted by the National Academy of Sciences’ National Research Council of biological and epidemiological data on the health risks of exposure to ionizing radiation.5 These data were derived from atomic bomb survivors, populations exposed during accidental releases of radioactive materials, workers with occupational exposures, and patients exposed to radiation during diagnostic studies and therapeutic procedures. Based on these data, the Institute of Medicine deduced that a single CT scan can result in exposures that are equivalent to those of long-term Hiroshima and Nagasaki survivors who were found to have increased lifetime risks of solid cancers and leukemia. Because of these risks, those who undergo repeated radiation exposure are typically monitored and restricted by government regulatory agencies to effective doses of 100 mSv every 5 years, or 20 mSv annually with a maximum of 50 mSv in any given year.6,7
Unfortunately, patients who undergo diagnostic imaging are not regularly monitored. In this study, we attempt to quantify the amount of radiation to which a typical trauma patient is exposed. We hypothesized that trauma patients would, by necessity, be exposed to high levels of ionizing radiation in the diagnosis of potential injuries.
METHODS
We studied all patients who met emergency department triage criteria for evaluation by the trauma service of Lancaster General Hospital from July 1, 2008 to June 30, 2009. Lancaster General Hospital is a Pennsylvania Trauma System Foundation (PTSF) accredited Level II Trauma center with a 95% to 5%, blunt to penetrating ratio. The trauma service consists of fellowship-trained and community general surgeons who evaluate and treat these patients.
Data collected retrospectively for each patient included age, Injury Severity Score (ISS), and the diagnostic CT scans they received. The information regarding diagnostic scans was derived from procedural codes associated with CT imaging. The estimated effective doses for each CT scan performed were based on the published literature and are listed in Table 1. The approximate total radiation exposure for each patient was the sum of the radiation exposures associated with all CT scans performed on that patient.
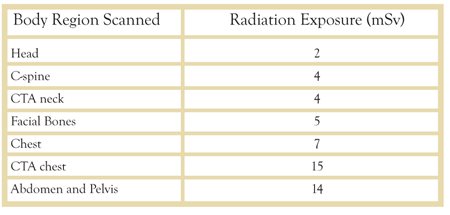
Table 1: Typical Effective Doses of Radiation Exposure of Common Computed Tomography Scans by Body Region (mSv = milliSieverts)
Patients were sorted into four categories based on their radiation exposure:
1) No CT radiation exposure;
2) Low exposure: <5mSv;
3) Moderate exposure: 5-20mSv;
4) High exposure: >20mSv.
These results were tallied and tabulated. A two sample t-test was performed to determine statistical differences between the high group and the other categories with respect to age. Additionally, radiation exposure for each patient was plotted against their respective Injury Severity Score and analyzed for correlative significance using the Pearson correlation coefficient. Significance was measured at the p<0.05 level.
RESULTS
During the study period from July 2008 to June 2009, 2,237 patients were evaluated by the trauma service. The mean (±SD) age and Injury Severity Score of the trauma patients were 46.4 (±25.0) years and 9.7, respectively. Of the 2,237 patients evaluated, 358 (17%) had no exposure and 1,879 (83%) underwent at least one diagnostic CT scan. A total of 6,299 CT imaging studies was performed, or a mean of 2.77 (±1.67) studies per patient. The mean effective dose of radiation exposure was 17.8 mSv (±11.7) per person.
The 1,879 patients were sorted into the aforementioned categories based on their radiation exposure. 154 patients (7%) received low exposure, 414 (18%) received moderate exposure, and 1311 (58%) receiving high exposure. There was no statistically significant difference in age among the groups (p=0.81).
In order to determine if there is a statistical relationship between radiation exposure and ISS score, a scatterplot was created. The Pearson correlation coefficient was 0.098, indicating that there was no significant relationship between radiation exposure and severity of injury in our cohort of patients (Figure 1).
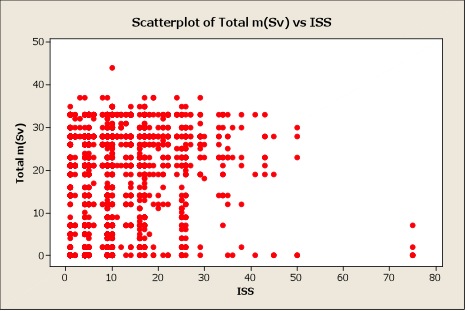
Figure 1. Correlational Scatterplot of Injury Severity Score (ISS) vs. Total Radiation Exposure in milliSieverts (mSv).
DISCUSSION
Computed Tomography scans have become instrumental in the diagnostic evaluation of patients since their introduction more than thirty years ago. As the technology improved with the introduction of spiral CT scanners, their use increased further. Additional improvements in speed and resolution have led to newer indications and lower thresholds for the use of CT imaging, and the approximate number of annual CT scans in the US has reached 70 million.8 In trauma patients, CT scans have become a frequent modality for the evaluation of the multiple-injured patient because they allow expeditious diagnosis and treatment. They can be used to identify and evaluate traumatic brain injuries, thoracic aortic injuries, solid organ abdominal injuries, and intra-articular fractures.
The speed of current CT scanners allows scanning of larger regions of the patient in the same time that previously was needed to perform selective CT scanning, which should result in fewer missed injuries, but multiphase studies mean higher radiation doses.2,9 In sum, the increased use of CT scans and the increased dose range per scan has created a steep rise in the patient’s exposure to ionizing radiation.
There are two types of risks associated with radiation, deterministic and stochastic. Deterministic risks are those with a predictable effect directly related to the quantity of radiation exposure, such as radiation burns. Stochastic risks are those associated with the effects of chance mutations which occur at random but are based on the level of radiation exposure. With increased exposure the probability of these mutations is increased, possibly resulting in radiation-induced cancers.10,11 Current models of stochastic risks suggest a linear relationship between dose and biological effect with no safe threshold. The overall detrimental biological effect of radiation exposure is designated the “effective dose.” The effective dose is calculated by weighting the concentration of energy left in each organ after an exposure to radiation with the use of parameters that emulate the type of radiation and the potential for radiation-associated mutagenic changes in a reference subject.12,13
Radiation exposure due to medical imaging is typically associated with stochastic carcinogenic risks, particularly the development of leukemia, thyroid and some solid organ cancers, as well as long-term risks of cataracts, sterility, and birth defects.11,14 Due to these risks, people that are likely to have repeated exposure to radiation are typically monitored and restricted to ensure that they are not overexposed to high levels. Regulations limits these persons to effective doses of 100 mSv every 5 years, on average 20 mSv per year, and a maximum of 50 mSv in any given year. The fields that most typically require this type of monitoring are health care and the nuclear industry.6
Health care workers involved in the resuscitation of trauma patients may be exposed to radiation during the initial radiographs taken as part of the resuscitation, and/or during adjunctive studies.15 Their level of exposure depends on the number of films, imaging modality, and distance to the radiation source. Due to the number of work-hours, residents in training are exposed to more radiation than nurses and emergency physicians.16 On average, residents, nurses, and emergency physicians received 0.20 (±0.28), 0.035 (±0.02), and 0.033 (±0.02) mSv per year, respectively. As the cited study was performed with dosimeters outside of lead garments, the reported annual doses would approximate the effective doses that unprotected persons would receive. Nurses receive a greater amount of radiation than others, presumably secondary to the proximity to the field of irradiation while performing duties. Even so, though exposure of workers in healthcare and the nuclear industry can be regulated, the amount of exposure of patients has not been restricted.
In 1990, the report from National Academy of Sciences on the Biological Effects of Ionizing Radiation (BEIR) V established an estimated lifetime attributable risk (LAR) of 0.8% for patients exposed to doses of 100 mSv.14 This estimate, as well as most other published estimates, is based on the results of the Life Span Study of survivors of Hiroshima and Nagasaki. More recently in 2006, further study of the survivors of the nuclear catastrophes was published in the BEIR VII report with the principal finding that exposure to 100 mSv would result in a solid organ cancer or leukemia in 1 in 100 persons, specifically 0.01 cancers per 100 mSv, again based on a linear non-threshold model for radiation exposure risk.5 Also, cancer may be more likely to develop in women than in men after similar levels of exposure.17
An analysis of age and sex-specific scan frequencies in the U.S. for 2007 in conjunction with the BEIR report was used to estimate the mean number of radiation induced cancers.18 Based on that analysis, Berrington de Gonzalez and colleagues concluded that approximately 29,000 future cancers could be related to the radiation exposure attributable to the CT scans performed during the year 2007, 66% of which were in females. CT scans of the abdomen, chest, and head would account for 14,000 cancers, 4,100 cancers, and 4,000 cancers, respectively. In another study, Smith-Bindman et al. noted large variations in radiation exposures from institution to institution with commonly performed CT scans,9 so they used mean dosages for the common scans to calculate potential cancer risk for men and women in three separate age ranges. Young women have the greatest lifetime attributable risk (LAR) of cancer due to radiation exposure; a 20 y/o female having a routine head CT scan (2 mSv) would have a LAR of 0.23 cancers per 1000 patients, or 1 cancer per 4,348 women receiving a CT scan of the head alone. That same 20 y/o female would have a LAR of 4 cancers per 1000 for a single multiphase CT scan of the abdomen and pelvis (31 mSv). Based on these data, the average annual exposure limit of 20 mSv would result in the development of one radiation induced cancer for every 150 women, age 20.
In order to help quantify how pervasive radiation exposure occurs from medical imaging procedures, Fazel et al. identified nearly one million patients in five health care markets during the study period of which 68.8% underwent at least one imaging procedure associated with radiation exposure.12 The mean (±SD) cumulative effective dose per enrollee per year was 2.4 (±6.0) mSv; however, the more alarming results are that approximately 194 out of every 1000 enrollees were exposed to moderate (3-20 mSv) doses and 20 out of every 1000 were exposed to more than 20 mSv. This study population was derived from hospitals, outpatient facilities, and physician offices under an individual health plan.
In the present study, our population is much more specific as the patients were triaged to require evaluation by the trauma service based on mechanism of injury and physiologic criteria. This population of patients is much more likely to undergo diagnostic imaging by CT scans, which are an adjunctive part of ATLS protocol.1 When comparing our results to the results published by Fazel et al., the trauma population is not only more like to receive radiation exposure (83% vs. 69%), but is more likely than the above described population to have high effective doses (>20 mSv) of radiation exposure (58% vs. 2%). This is an estimate based on typical published doses of CT scans.
Trauma patients in a study at the University of Pennsylvania had a mean ISS of 32.3±15.0, underwent an average of 70.1±29.0 radiographic studies, and had a cumulative dose of 106±56 mSv.19 These patients were the most severely injured, with ICU stays greater than 30 days (average 2 mo). CT scans constituted less than 10% of the total number of studies performed but accounted for two-thirds of radiation doses.
Investigators at Sunnybrook Health Sciences Centre in Toronto placed dosimeters on the neck, chest, and groin of all major trauma patients who required admission to the trauma service.20 These patients had a mean ISS of 22.7 and were found to have been exposed to 22.7 mSv of effective radiation dose. Additionally, 22% of these patients had thyroid doses greater than 100 mSv.
Whole Body Imaging
Computed tomography has been integral in the evolution of the management of trauma. It has allowed for non-operative management of solid organ injuries, early diagnosis of arterial injuries in the pelvis or thoracic aorta, and establishment of priorities by characterizing multi-system injuries. It aids in the identification of patients who might require inpatient observation or are candidates for early discharge. In many centers including ours, most team resuscitations receive at least one CT scan (83% in our institution). However, this liberal usage of scans may be detrimental to our community contributing to the epidemic of medical radiation exposure.
There have been several studies to suggest that whole body imaging (i.e. “pan-scan”) is justifiable and should be encouraged. Salim and colleagues at Los Angeles County Medical Center analyzed pan-scans done on 1,000 patients during an 18-month period.21 592 met inclusion criteria, i.e. had no visible evidence of injury in the torso, were hemodynamically stable, had an abdominal exam that was normal or could not be evaluated, and had a significant mechanism of injury. With this liberal use of the pan-scan, 189 patients required a change in treatment plan based on abnormal CT scan findings.
One of the limitations of the study was their inclusion criterion of “no visible injury.” This requirement did not take into account palpatory or subjective findings outside the area of the abdomen which would then warrant further investigation. Another limitation involves the definition of change in treatment (early discharge, admission for serial exams, additional diagnostic studies, and immediate operative intervention) and whether the need for immediate operative intervention would have become manifest in due course.
In another recent study by Tillou and colleagues, 284 patients with injuries from blunt trauma who underwent pan-scanning were evaluated for the necessity of each CT radiograph.22 (Note that “pan scanning” is not a single scan, but rather it consists of scans of the brain, c-spine, chest, abdomen/pelvis, and possibly more.) Of the 311 scans deemed unnecessary by a physician, 52 scans were positive for some type of injury. Of the 52 positive scans, two required immediate intervention. This article concludes there is potential to miss injuries in 17% of patients of patients who did not undergo whole body imaging. One of the major flaws is that all but 15 of the greater than 1000 scans deemed unnecessary were deemed so by emergency room physicians not trauma surgeons. Further experience or difference in training might account for the discrepancy.
Some experts state that the potential increased risk of cancer due to radiation exposure is based on inaccurate models. The so-called linear, no-threshold theory used by national and international committees as a basis to provide radiation protection recommendations overestimates cancer risk, and it is actually much lower. These same experts believe there is no radiation-related cancer risk as long as the exposure to low-dose radiation is below a certain threshold.23 However, without use of such models, the only truly accurate method to quantify the risks from CT scans would be direct long-term follow-up of very large populations.24
Our study at LGH shows that without specific measures to curb pan-scanning, whether justified or not, the majority of trauma patients receive greater than the average annual allowable effective dose of ionizing radiation. There are two methods to decrease the amount of radiation exposure: 1) to optimize the indications for CT imaging, thus ensuring that exposure is as low as reasonably achievable (ALARA) without sacrificing the quality of care; and 2) to maximize protective strategies such as optimization of CT dosage for all scanners and use of effective shielding.
Although there are many protocols to reduce the radiation exposure of children undergoing CT scans, a majority of the protocols are specific to individual CT scanners. Currently at Lancaster General Health, the CT scanner being used provides adaptive scanning. The topogram allows measurement of the posterior-anterior distance of each individual patient, and this information can be used to determine the minimal amount of power necessary to obtain proper penetration for the necessary images. The amount of radiation delivered is thus adapted to that individual patient for that particular exam.
Additionally, policies and protocols in place at LGH attempt to minimize radiation exposure with respect to the examination to be performed. This includes organ specific protocols that adhere to the principle of ALARA; shielding vulnerable body regions from radiation scatter whenever appropriate; and focused review of any cases of high risk radiation exposure by the Radiation Safety Officer.
CONCLUSIONS
This study is presented to bring attention to medical imaging and radiation exposure as a true concern in health care. Trauma is a greater concern than other medical conditions in the younger population, and unfortunately, the young are most affected by exposure to ionizing radiation. With a majority of trauma patients receiving high levels of radiation with no significant correlation with injury severity score, we, the decision makers in trauma, must make judgments based on history and physical exam to utilize imaging appropriately. The Alliance for Radiation Safety in Pediatric Imaging has a campaign entitled Image GentlySM. As physicians dealing with trauma patients at ground zero of radiation exposure, we should extend that concept to include Image Gently and Appropriately.25
REFERENCES:
1. American College of Surgeons – Committee on Trauma. Advanced trauma life support course for physicians: ATLS. 8th ed. Chicago (IL): The College; 2008.
2. Amis ES Jr, Butler PF, Applegate KE, et al. American College of Radiology. American College of Radiology white paper on radiation dose in medicine. J Am Coll Radiol. 2007;4(5):272-284.
3. IMV Medical Information Division. CT Census Database and Market Summary Report. Greenbelt, MD: IMV;2008.
4. Mettler FA Jr, Huda W, Yoshizumi TT, et al. Effective doses in radiology and diagnostic nuclear medicine: a catalog. Radiology. 2008;248(1):254-263.
5. National Research Council of the National Academies. Health Risks from Exposure to Low Levels of Ionizing Radiation: BEIR VII Phase 2. Washington, DC: National Academies Press; 2006.
6. The 2007 recommendations of the International Commision on Radioloogical Protecction: ICRP publication 103. Ann ICRP 2007;37(2-4):1-332.
7. Wrixon AD. New ICRP recommendations. J Radiol Prot 2008;28:161-168.
8. Mettler FA Jr, Thomadsen BR, Bhargavan M, et al. Medical radiation exposure in the US in 2006: preliminary results. Health Phys 2008; 95(5):502-507.
9. Smith-Bindman R, Lipson J, Marcus R, et al. Radiation dose associated with common computed tomography examinations and the associated lifetime attributable risk of cancer. Arch Intern Med. 2009 Dec 14;169(22):2078-2086.
10. Brenner D, Elliston C, Hall E, et al. Estimated risks of radiation-induced fatal cancers from pediatric CT. AJR Am J Roentgenol 2001;176:289-296.
11. Ron E. Ionizing radiation and cancer risk: evidence from epidemiology. Pediatr Radiol. 2002;32:232-237.
12. Fazel R, Krumholz HM, Wang Y, et al. Exposure to low-dose ionizing radiation from medical imaging procedures. N Engl J Med. 2009 Aug 27;361(9):849-857.
13. Martin CJ. The application of effective dose to medical exposures. Radiat Prot Dosimetry 2008;128:1-4.
14. National Research Council of the National Academies. Health Risks from Exposure to Low Levels of Ionizing Radiation: BEIR V. Washington, DC: National Academies Press; 1990.
15. Tan GA, Van Every B. Staff exposure to ionizing radiation in a major trauma centre. ANZ J Surg 2005;75:136-137.
16. Ciraulo DL, Marini CP, Lloyd GT, et al. Do surgical residents, emergency medicine physicians, and nurses experience significant radiation exposure during the resuscitation of trauma patients? J Trauma 1994;36:703-705.
17. Einstein AJ, Henzlova MJ, Rajagopalan S. Estimating Risk of Cancer Associated With Radiation Exposure From 64-Slice Computed Tomography Coronary Angiography. JAMA 2007;298:317-323.
18. Berrington de González A, Mahesh M, Kim KP, et al. Projected cancer risks from computed tomographic scans performed in the United States in 2007. Arch Intern Med. 2009 Dec 14;169(22):2071-2077.
19. Kim PK, Gracias VH, Maidment AD, et al. Cumulative radiation dose caused by radiologic studies in critically ill trauma patients. J Trauma 2004;57:510-4.
20. Tien HC, Tremblay LN, Rizoli SB, et al. Radiation exposure from diagnostic imaging in severely injured trauma patients. J Trauma 2007:62:151-6.
21. Salim A, Sangthong B, Martin M, et al. Whole body imaging in blunt multisystem trauma patients without obvious signs of injury: results of a prospective study. Arch Surg 2006;141(5):468-73; discussion 473-5.
22. Tillou A, Gupta M, Baraff LJ, et al. Is the use of pan-computed tomography for blunt trauma justified? A prospective evaluation. J Trauma. 2009 Oct;67(4):779-87.
23. Tubiana M, Feinendegen LE, Yang C, Kaminski JM. The linear no-threshold relationship is inconsistent with radiation biologic and experimental data. Radiology. 2009; 251(1):13-22.
24. Land CE. Estimating cancer risks from low doses of ionizing radiation. Science. 1980;209(4462):1197-1203.
25. Image Gently [Pediatric Radiology Alliance for Radiation Safety in Pediatric Imaging Web site]. Available at http://www.pedrad.org/associations/5364/ig/ Accessed Sept 20, 2010.