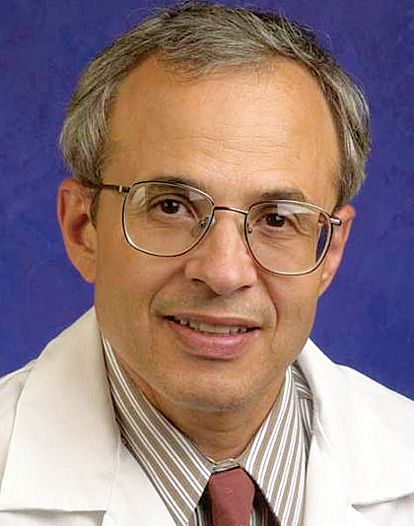
Spring 2021 - Vol. 16, No. 1
The Diaphragm:
The Forgotten Factor in Ventilator Weaning
Robert L. Vender, M.D.
Director of Pulmonary, Allergy and Critical Care Medicine
Penn State Health Milton S. Hershey Medical Center
PROLONGED MECHANICAL VENTILATION: IMPORTANCE OF THE DIAPHRAGM
Although patients who require prolonged mechanical ventilation (PMV) comprise less than 10% of all intensive care unit (ICU) patients, they represent one of the most rapidly growing ICU populations, and can utilize up to 50% of ICU resources.
1 Even with the best of care, they experience a 75% mortality at 1 year, and rarely resume functioning at a high level.
2,3
Patients who remain critically ill for prolonged periods are usually characterized by their requirement for PMV, whether continuous endotracheal intubation and invasive mechanical ventilator support for greater than 21 days, or a need for tracheostomy in anticipation of PMV.
4
The initial events that precipitate
acute respiratory failure are rarely the same as the factors that cause a need for
continuous mechanical ventilation. Common factors that lead to ventilator dependency are reduced mental status including delirium, and – more often – failure of inspiratory muscles, predominately the diaphragm.
The diaphragm is often the forgotten factor in weaning and liberation from a mechanical ventilator. All ICU patients who require invasive mechanical ventilation are at risk of developing the need for PMV, but most current treatment practices focus on treating complications
after they become overt, rather than preventing them. Treatment strategies often fail to identify therapies that prevent PMV, or fail to improve success rates for liberation from mechanical ventilation.
Nevertheless, there do appear to be potential modifiable factors, which – if addressed proactively – could assist in resolving this growing problem. Recent studies and expert opinions have generated two important paradigms in acute ICU management of mechanical ventilators which could have a major impact in preventing clinical progression to PMV. These include:
1) identifying multiple causes of diaphragm dysfunction, termed diaphragm myo-trauma, and
2) using ventilation strategies that protect both lung and diaphragm.
5,6
Although discussed separately, both of these new concepts are integrally linked.
SUSTAINED SPONTANEOUS VENTILATION: BACKGROUND AND PRINCIPLES
As background, it should be understood that the ability to maintain sustained spontaneous ventilation is analogous to the concept of repetitive muscular training exercises in the gymnasium (i.e. “reps”). If the load is excessive (too heavy), the frequency (rate) is too high, and/or muscle strength is too weak, then task fatigue/failure will develop. Survival is dependent upon the endurance capacity of the inspiratory muscles (the diaphragm being predominant) to overcome the “weight” of each spontaneous breath.
While ordinary skeletal locomotor muscles work to overcome inertia, the skeletal muscle diaphragm must work with the intercostal muscles to overcome the forces that comprise the work of breathing, which encompass:
1) the elastic load of inflation of the lungs and expansion of the chest wall, and
2) the non-elastic load of airway resistance. In health, the diaphragm is well positioned both anatomically and physiologically to achieve the balance needed for effective sustained spontaneous ventilation.
During restful tidal breathing, the diaphragm is in active contraction at most only approximately 20% of the time; it utilizes only 10%-12% of its maximal capacity; and it consumes only 1%-2% of the body’s total oxygen consumption. Respiratory failure ensues in disease states when an imbalance develops between the lung “load” and the diaphragm’s endurance. Although contemporary ICU practices focus mostly on the lung (i.e. the increased work of breathing), equal attention should also be given to maintaining the diaphragm’s strength, tension, endurance, and contractility, in anticipation of potentially successful transition from dependence on the ventilator to spontaneous ventilation.
VENTILATOR-INDUCED DIAPHRAGMATIC DYSFUNCTION
An expanded understanding of the multiple ventilator-induced mechanisms of diaphragmatic myo-trauma has generated several hypothetical ventilator management recommendations. These are now being validated by:
a) tissue Doppler ultrasound imaging of the diaphragm, and
b) classic physiological assessments. These diagnostic modalities are designed to assess the balance between the capability of the inspiratory muscles (most specifically the diaphragm), and the work of breathing, not only to predict the likelihood of weaning, but more importantly to identify treatment and management concepts that could prevent myo-trauma from becoming overt.
The different mechanisms of diaphragmatic myo-trauma include:
1) Over-assistance ventilation resulting in disuse atrophy and/or sarcomere proteolytic degradation,
2) Under-assistance ventilation resulting in contractile fatigue secondary to excessive load,
3) Eccentric myo-trauma from diaphragmatic contraction in the midst of lengthening such as occurs during reverse triggering, and
4) Expiratory myo-trauma when the diaphragm is maintained at shortened length, often due to excessive positive end expiratory pressure (PEEP) leading to sarcomere dropout.
5
Standard ventilator management strategies in the ICU are designed to prevent ventilator induced lung injury (VILI), and therefore include monitoring of respiratory variables (peak airway pressure, plateau airway pressure, tidal volume, and inspired concentrations of oxygen). However, to protect both the diaphragm and the lung during mechanical ventilation, it is equally important to monitor respiratory effort as per the “lung-diaphragm protective ventilation” strategy.
6
TISSUE DOPPLER ULTRASOUND OF THE DIAPHRAGM (TDU)
TDU of the diaphragm has emerged as a potentially valuable tool not only to monitor its muscularity and contractility, but also to guide the weaning process. Specific TDU of the diaphragm measurements included
1) a measure of musculature – quantification of thickness at end inspiration and end expiration,
2) an index of contractility – the extent and distance of shortening during contraction (i.e. excursion), and
3) an indicator of dynamics – the velocity of shortening.
The percent change in diaphragm thickness (Tdi
*) between end-expiration and end-inspiration (∆Tdi%) is calculated as [Tdi end-inspiration – Tdi end-expiration/ Tdi end-expiration] X 100. In critically ill patients breathing spontaneously, or undergoing trials of weaning from pressure support in anticipation of extubation, a change in diaphragm thickening fraction during contraction (∆Tdi%) of greater than 30% predicted weaning success with 88% sensitivity.
7 The authors noted that thickening of the diaphragm during inspiration reflects shortening of the diaphragm and is analogous to the heart’s ejection fraction.
7 TDU assessment of the diaphragm’s dynamic activity has also identified peak contraction velocity (PCV) as a parameter that may be useful in predicting weaning success or failure. Measured values for PCV of 1.36 cm/sec seem predictive for extubation success and PCV values in excess of 2.02 cm/sec predictive of failure.
8
ICU APPLICATIONS OF CLASSIC PHYSIOLOGICAL ASSESSMENTS OF THE DIAPHRAGM'S ENDURANCE
In addition to contemporary utilization of TDU, certain physiological measures of respiratory effort have been validated as components of the ventilation paradigm that can be monitored to maximize lung-diaphragm protection:
(a) Tension time index (TTi),
(b) Diaphragmatic pressure time product (PTPdi),
(c) Airway occlusion pressure at 100msec (P0.1).
Both TTi and PTPdi are accurate, objective, quantitative measures of inspiratory muscle endurance. The TTi and PTPdi utilize measurements of trans-diaphragmatic pressure (Pdi) [Pgastric – Pesophageal], plus the duty cycle of each breath.
The duty cycle of each breath is defined as inspiratory time (Ti) divided by total breath cycle time (Ttot), which includes inspiration and expiration. The resulting fraction (Ti/Ttot) represents the time that the diaphragm is maintained in active contraction. Any circumstance that increases the diaphragm’s duty time will hasten the development of load failure and fatigue.
In healthy subjects, maximal Pdi (Pdimax) measurements approximate 100 ± 25 cm H
2O with Pdi/Pdimax during tidal restful breathing approximating 20%. Of note regarding sustained spontaneous ventilation, Pdi/Pdimax >40% cannot be maintained indefinitely even by healthy individuals.
(a) TTi is calculated as Ti/Ttot X Pdi/Pdimax. At rest, healthy subjects maintain TTi of approximately 0.02; in stable patients with COPD, TTi values equal a mean of 0.05. Studies suggest that TTi < 0.15 can be maintained indefinitely while TTi > 0.18 leads to fatigue; i.e. the threshold of fatigue would be between 0.15 and 0.18.
9
(b) The PTPdi is the pressure analog of TTi, calculated as the area under the Pdi vs. Time inflation curve. Normal values are PTPdi < 5 cm H
2O.sec/breath, or < 50 cm H
2O.sec/min. Values greater than 200 cm H
2O.sec/min are indicative of excessive ventilatory muscle effort and predict failure to extubate.
8,10,11
The airway occlusion pressure (P0.1) is the drop in airway pressure (Paw) 100 milliseconds (0.1 sec) after onset of inspiration during an end-expiratory occlusion of the airway as measured most commonly with an esophageal balloon. Normal values for healthy subjects equal 0.7 cmH
20 (range 0.6-1.0). In theory P0.1 provides a reliable measure of central nervous system respiratory drive because the brevity of airway occlusion means it is:
1) independent of respiratory mechanics,
2) too rapid to be sensed via peripheral neurosensory feedback (insufficient time for behavioral response to influence pressure output of the inspiratory muscles), and
3) not influenced by respiratory muscle weakness to a certain degree.
In patients requiring invasive mechanical ventilation the P0.1 correlates closely with PTPdi/min, with P0.1 > 3.5 cm H
20 correlating with PTPdi/min > 200 cmH
2O.sec/min. Both accurately predict extubation failure resulting from diaphragm fatigue due to work overload.
11
SUPPORTIVE DATA AND EXPERT RECOMMENDATIONS
Based upon these principles there exist three lines of data in support of a ventilation strategy that protects the diaphragm:
a) animal experimental data,
b) ongoing active human clinical studies, and
c) expert opinion and recommendations.
An experimental animal study of sedated and mechanically ventilated pigs for 2.5 days demonstrated that catheter-based trans-venous phrenic nerve pacing mitigated ventilator-induced diaphragm atrophy. The latter was assessed by both ultrasound measurement of the diaphragm’s thickness, and muscle biopsy of the diaphragm for histological quantification of muscle fiber cross sectional area and myofiber size.
12 Similar current active ongoing human clinical studies are in progress using similar methods of catheter based phrenic nerve stimulation in attempts to prevent and/or ameliorate diaphragmatic disuse atrophy.
This expanded understanding has generated expert opinion and recommendations for mechanical ventilator modes designed to target diaphragm protection. In general, these recommendations include:
1) Maintaining modest spontaneous inspiratory effort,
2) Maintaining ventilator-patient synchrony, and
3) Paying attention to the expiratory phase of ventilation.
When feasible, the specific and practical ventilator management parameters now recommended for optimum protection of the diaphragm include:
1) Prevention of over-assistance myo-trauma (i.e. patient not working enough) to be achieved with any one of the following targets assessed during inspiratory effort:
a) ∆Pdi > 3–5 cm H
2O,
b) ∆Pes (esophageal pressure) < –2 to –3 cm H
2O,
c) P0.1 > 1–1.5 cmH
2O,
d) ∆Tdi > 15%;
2) Prevention of under-assistance myo-trauma (i.e. patient working too much) with any one of the following targets assessed during inspiratory effort:
a) ∆ Pdi < 10–15 cm H
2O,
b) ∆Pes > –8 to –12 cm H
2O,
c) P0.1 < 4 cm H
2O,
d) ∆Tdi < 30–40%; and
e) TTi < 0.12
3) Prevention of eccentric myo-trauma by avoiding ineffective triggering and/or reverse triggering.
6
CONCLUSIONS
Prolonged mechanical ventilation (PMV) is frequently a consequence of muscle disease, particularly of the diaphragm, and does not always result from lung disease alone.
Monitoring of lung physiology and the effort of respiratory muscles and the diaphragm are essential components of the “lung-diaphragm protective ventilation” strategy. Monitoring methods include both Tissue Doppler Ultrasound and/or basic physiology measurements.
The potential clinical benefit of these principles and recommendations has yet to be determined.
REFERENCES
1. Nelson JE, Cox CE, Hope AA, et al. Chronic critical illness.
Am J Respir Crit Care Med. 2010; 182: 446-454.
2. Daly BJ, Douglas SI, Gordon NH, et al. Composite outcomes of chronically critically ill patients 4 months after hospital discharge.
Am J Crit Care. 2009; 18: 456-464.
3. Unroe M, Kahn JM, Carson SS, et al. One-year trajectories of care and resource utilization for recipients of prolonged mechanical ventilation: A cohort study.
Ann Inter Med. 2010; 153: 167-175.
4. Vender RL, Vender LM. The chronic critically ill: pulmonary perspective.
J Nurs Care. 2015; 4(2): doi 10.4172/2167-1166.1000239.
5. Goligher EC, Brochard LJ, Reid WD, et al. Diaphragmatic myotrauma: a mediator of prolonged ventilation and poor patient outcomes in acute respiratory failure.
Lancet Respir Med. 2018; 7: 90-98..
6. Goligher EC, Dres M, Patel BK, et al, for The Pleural Pressure Working Group, Acute Respiratory Failure Section of the European Society of Intensive Care Medicine. Lung and Diaphragm-Protective Ventilation.
Am J Respir Crit Care Med. 2020; 202(7): 950-961.
https://doi.org/10.1164/rccm.202003-0655CP
7. DiNino E, Gartman EJ, Sethi JM, et al. Diaphragm ultrasound as a predictor of successful extubation from mechanical ventilation.
Thorax. 2014; 69: 423-427.
8. Soilemezi E, Savvidou S, Sotiriou P, et al. Tissue doppler imaging of the diaphragm in healthy subjects and critically ill patients.
Am J Respir Crit Care Med. 2020; 202(7): 1005-1012.
9. Mador MJ. Respiratory muscle fatigue and breathing pattern.
Chest. 1991; 100: 1430-1435.
10. Rittayamai N, Beloncle F, Goligher EC, et al. Effect of inspiratory synchronization during pressure-controlled ventilation on lung distension and inspiratory effort.
Ann Intensive Care. 2017; 7: 100. Doi
10.1186/s13613-017-0324-z.
11. Telias I, Juhnasavasdikul D, Rittayamai N, et al. Airway occlusion pressure as an estimate of respiratory drive and inspiratory effort during assisted ventilation.
Am J Respir Crit Care Med. 2020: 201(9): 1086-1098.
12. Reynolds SC, Meyyappan R, Thakkar V, et al. Mitigation of ventilator-induced diaphragm atrophy by transvenous phrenic nerve stimulation.
Am J Respir Crit Care Med. 2017; 195(3): 339-348.
* In this and subsequent formulas, “di” denotes a value for a property of the diaphragm, e.g. Pressure (P) or Time (T), whereas the letter “i” standing alone denotes an index of a value.