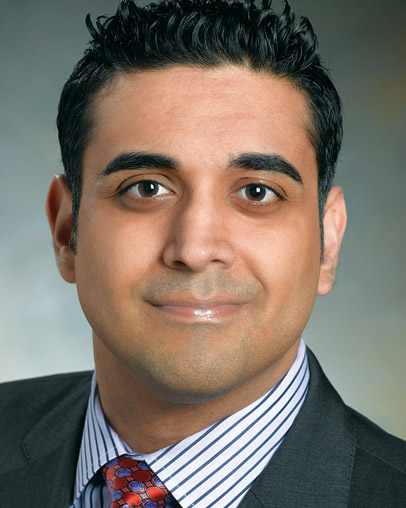
Winter 2017 - Vol. 12, No. 4
Cardio-Oncology: An Integrated
Discipline or Just a Portmanteau?*
INTRODUCTION
Cardio-oncology is a rapidly growing and evolving field that aims to optimize the cardiovascular (CV) care received by cancer patients before and after chemotherapy. There are now more than 14.5 million cancer survivors in the United States, and it is estimated that by the year 2020, there will be roughly 18 million.
1
There is a broad range of cardiotoxic side effects of chemotherapy and radiation treatment (Fig. 1).
Fig. 1.
Cardiotoxic Side Effects of Chemotherapy and Radiation Treatment
Source: Lenneman CG, Sawyer DB. Cardio-Oncology: An update on Cardiotoxicity of Cancer-Related Treatment. Circ Res 2006;118: 1008-1020.
Cardiotoxicity used to be considered just a reduction in resting left ventricular ejection function (LVEF), but it is now understood to include direct effects on cardiac structure and function, the cardiac conduction system, the systemic and pulmonary vasculatures, hemostasis and thrombosis, as well as direct injury and stress to the endothelium. As a result of the latter, coronary artery disease (CAD) is second only to recurrence of cancer as a leading cause of death in cancer survivors.
Cardio-oncology programs are thus essential to identify risk factors for cardiotoxicity, to treat and reduce them, and to monitor for CV toxicities from prior and ongoing chemo- and radio-therapy regimens. The goal is to optimize cancer patients prior to chemotherapy, to help identify cardiotoxicities early, and to institute pharmacotherapy when necessary, in hopes of reducing morbidity and mortality from cardiac events without impeding or disrupting oncology treatment regimens.
THE BASELINE CLINICAL ASSESSMENT
In the cardio-oncology clinic, the risk of an individual patient developing cardiotoxicity from treatment is assessed well before chemotherapy begins. The two key factors are: 1) the specific chemotherapy and/or radiation therapy proposed, and 2) coexisting CV disease, age and sex. Especially in older patients, pre-existing conditions may limit therapeutic options, so it is imperative prior to starting chemotherapy to risk-stratify patients, to optimize blood pressure, lipids, and blood sugar, and to encourage tobacco cessation. Patients found to have a subnormal LVEF should be started on goal-directed medical therapy for congestive heart failure to optimize heart function. The cardiologist and oncologist should collaboratively consider whether cardiotoxic chemotherapy is in the best interest of the patient. Patients at highest risk may benefit from cardioprotective medical therapy with beta-blockers, statins, angiotensin converting enzyme (ACE) inhibitors, or angiotensin receptor blockers (ARB).
2
Interestingly, many of the risk factors associated with the development of CV disease, such as tobacco use, are also risk factors for developing cancer. It is postulated that inflammation not only increases the risk of malignancy, but is the common denominator that links the development of CV diseases, diabetes, atherosclerosis, endothelial dysfunction, and metabolic syndrome. High C-reactive protein (CRP) levels are associated with an increased incidence of CV events and an increased risk of developing a malignancy, and a higher CRP portends worse survival.
1 In regard to the interactions between various molecular pathways that are not yet fully elucidated, it is relevant that the same risk factors for coronary atherosclerosis also increase the risk of CV complications from chemotherapy.
A baseline cardiac assessment should be performed on patients with the following cardiac risk factors: left ventricular (LV) dysfunction, age<18 or >65, planned chemotherapy with multiple cardiotoxic agents or high doses of a single cardiotoxic drugs, known prior cardiac pathology (LV hypertrophy, hypertension, CAD), diabetes mellitus or prior radiation exposure.
1 Risks should be minimized and patients should be treated prior to chemotherapy; as feasible, patients may benefit from referral to a cardio-oncologist who specializes in treating and following cancer patients through survivorship. Nevertheless, the large and growing number of cancer patients makes it prudent for internists, generalists, cardiologists, oncologists, and hematologists to all become well versed in risk-stratifying and caring for these patients in regard to cardiotoxicity.
CARDIOTOXICITY
Over the last four decades, strategies aimed at detecting cardiac dysfunction have evolved from invasive, direct endomyocardial biopsies, to non-invasive cardiac imaging. Terminology has also evolved, and the term “Cancer Therapeutics Related (or Chemotherapy-Related) Cardiac Dysfunction (CTRCD) has been adopted to describe LV dysfunction related to cancer treatment, as well as the type of myocardial injury.
CTCRD is defined as a > 5% drop in LVEF in symptomatic heart failure (HF) patients, or a >10% drop in LVEF to <53% in the asymptomatic patient. There are two main types of CTRCD:
a) Type I is dose-dependent, irreversible, and results from ultra-structural changes within the myocardium. It is typified by the anthracycline class of drugs which inhibit function of topoisomerase 2B within cardiomyocytes, leading to DNA double strand breaks, mitochondrial dysfunction, and development of reactive oxygen species that lead to damage or death of the cardiomyocytes.
b) Type II is mostly reversible, is not dose-dependent, and is not associated with ultra-structural changes in the myocardium, so functional recovery is often seen after interrupting and discontinuing therapy.
Trastuzumab (Herceptin®) is a typical causative agent, but since it was approved, dozens of other chemotherapy agents have been introduced that also cause reversible cardiotoxicity, especially the small-molecule tyrosine kinase inhibitors (TKI) and vascular endothelial growth factor (VEGF) inhibitors. Their net effect on the CV system is still being studied and the cardiotoxicity rates are unpredictable. Attempts have been made to propose a unified approach to managing these complex patients, but there is no single, evidence-based, guideline for management, and the approach to treatment, evaluation, and drug stoppage is varied at best.
The incidence of cardiomyopathy, potential CV toxicities and current uses of common chemotherapeutic agents are summarized in Table 1.
Table 1.
The Incidence of Cardiomyopathy, Potential CV Toxicities, and Current Uses of Common Chemotherapeutic Agents
ANTHRACYCLINES
Cardiac dysfunction from cancer therapeutics was first widely recognized in the 1960s with a new class of chemotherapeutics—the anthracyclines – a type of antibiotic derived from Streptomyces bacteria. Though their use continues to be limited by cardiotoxicity, they are used for a variety of cancers including lymphoma, leukemia, sarcoma and breast cancer, and are still among the most effective chemotherapeutic agents.
3 Exposure to these drugs causes both short- and long-term complications and risk largely depends on the cumulative dose. At a cumulative dose of 400 mg/m
2, the risk of developing HF is around 5% but increases dramatically to about 25% when the cumulative dose reaches 700 mg/m
2.
4 A thorough assessment to identify underlying risk factors is essential, as patients with hypertension, CAD, diabetes, LV dysfunction, or prior radiation are at much higher risk for developing toxicity at much lower doses. These patients must be closely followed during and after therapy and the total cumulative dose must be appropriately adjusted, or the drug may have to be changed.
5,6 Acute cardiac dysfunction is reported in up to 3.2% of patients receiving anthracycline therapy and usually occurs within weeks of starting therapy. These patients usually present with arrhythmias, HF, LV dysfunction, and myocarditis-pericarditis syndrome, but occult LV dysfunction may be present in up to 20% of patients, and may not be clinically evident until the completion of chemotherapy. Late complications of anthracycline therapy include the development of HF decades after treatment.
The use of an encapsulated (“pegylated” by a liposomal moiety) form of
doxorubicin (Adriamicin
TM) allows a higher cumulative dose with a lower rate of myocardial damage, but with similar efficacy. Despite this, however, its use does not eliminate the advisability of routine surveillance of LV function during therapy.
Another approach is the use of
dexrazoxane, an iron chelator like EDTA that protects the myocardium from the effects of doxorubicin or epirubicin. These cardioprotective effects have been clinically confirmed in a number of studies, but there are concerns about a lower response rate and development of secondary leukemia in childhood survivors.
7 Epirubicin is less cardiotoxic than doxorubicin and in studies has been shown to decrease the risk of both clinical and subclinical cardiotoxicity.
7 Nevertheless, it is still cardiotoxic and the Food and Drug Administration has limited the cumulative dose to < 900 mg/m
2 per patient.
HER2 ANTAGONISTS
HER2/neu antagonists, typified by
trastuzumab (Herceptin®), are a relatively new type of chemotherapy for breast cancer – monoclonal antibodies that target and inhibit the Human epidermal growth factor receptor-2 (also known as ErbB2).
Trastuzumab has dramatically improved the formerly dismal prognosis of patients with tumors that overexpress the HER2 protein, which tend to be rapidly progressive, but cardiotoxicity is an ever-present risk.
In one meta-analysis, treatment with
trastuzumab significantly increased the incidence of congestive heart failure (RR 5.11, 90% CI [3.0-8.7]; P<0.000001) and the LVEF declined significantly (RR 1.83, 90% CI [1.36-2.47], P=0.0008).
8 In pre-clinical studies, cardiac dysfunction with HER2 targeted therapies occurred secondary to disruption in signaling between the HER2 receptor and the ligand growth factor, Neuregulin.
2 The HER2 (ErbB2)-Neuregulin receptor ligand-signaling cascade is critical to myocyte growth, survival and homeostasis, and HER2 targeted therapies interrupt myocyte homeostasis and cardiac repair by affecting the signaling pathway. Furthermore, this signaling pathway regulates vasomotor tone and sympathetic output within the CV system; breast cancer patients treated with HER2 antagonists experience increased levels of norepinephrine, heart rate and blood pressure.
2
Newer HER2 targeted therapies have emerged in the last decade including
lapatinib, an oral HER2 targeted small molecule, dual TKI of the Her2/neu and the epidermal growth factor receptors.
Pertuzamab, another HER2 targeted agent, was designed to overcome
trastuzumab resistance. Thus far, clinical studies with the newer HER2 targeted agents have not shown clear evidence for causing cardiotoxicity.
1 Routine monitoring of cardiac function during treatment with any HER2 antagonist has become standard practice and most expert panels, based on clinical studies, recommend echocardiograms every three months during therapy.
The novel HER2 antagonists have significantly improved outcomes in breast cancer patients, but we must identify high-risk patients, frequently monitor for the development of cardiotoxicity during and after therapy, and initiate HF therapies when appropriate.
ALKYLATING AGENTS
Cisplatin is a commonly used alkylating agent in several solid organ tumors (genitourinary, gastrointestinal, head and neck), and vascular toxicity is the primary concern. Given the high cure rates, long-term consequences post treatment are emerging, particularly CV diseases, including hypertension, dyslipidemia, early atherosclerosis, CAD, Raynaud’s phenomenon, and thromboembolic events. Testicular cancer survivors treated with
Cisplatin-based therapy have an increased risk of premature atherosclerosis, increased biomarkers suggestive of endothelial injury, and development of CAD and vascular disease.
1 Cisplatin is incompletely eliminated after treatment, and platinum can be measured in the serum years after therapy, leading to speculation that this continuous small amount of
Cisplatin leads to endothelial dysfunction.
Cyclophosphamide has also been associated with cardiotoxicity that is not clearly dose-dependent, including reduced LVEF, decreased electrocardiogram (ECG) voltage with or without pericardial effusions, and – most severely – hemorrhagic myopericarditis with pericardial effusion, attributed to endothelial capillary damage. This last complication can occasionally lead to cardiac tamponade, but otherwise these scenarios are managed conservatively. The risk of cardiotoxicity is increased by prior radiation therapy to the chest and mediastinum, older age, and reduced LVEF.
MULTI-TARGETED TKI AND VEGF INHIBITORS
Angiogenesis is a requirement for adequate tumor cell growth, and multiple anti-tumor therapies target the angiogenesis-signaling cascade. The first of these were inhibitors against VEGF and more recently small molecules that inhibit VEGF receptor tyrosine kinase and non-receptor tyrosine kinases. However, since VEGF plays an integral role in angiogenesis, endothelial cell survival, vasodilatation, and cardiac contractile function, alterations in angiogenesis inevitably impact CV biology and function. The small molecule receptor TKIs have an even more complex and variable CV effect on the cardiac system than do the monoclonal antibody based biologics.
VEGF also regulates endothelial cell health, survival, and repair; thus inhibition promotes microvascular injury and potentiates thrombosis. In addition to causing hypertension and cardiac dysfunction, VEGF-targeted therapies can promote thromboembolism and are associated with a three-fold increase in arterial thromboembolic events such as transient ischemic attacks, strokes, angina, and myocardial infarction.
VEGF signaling is also known to mediate adaptation of the myocardium to pressure overload. Suppressing VEGF during pressure overload leads to a rapid deterioration of heart function and promotes adverse cardiac remodeling. This phenomenon has been observed with
bevacizumab (an anti-VEGF monoclonal antibody) and with
sunitinib and a number of other multi-TKIs.
1 It is still unclear whether cardiac dysfunction secondary to multi-TKIs is reversible. Early studies with
sunitinib have shown that LV dysfunction and HF symptoms respond well to discontinuing the offending drug and starting goal directed HF therapies. However, pre-clinical animal and cellular data show
sunitinib can promote and induce cardiomyocyte apoptosis thus at least some element of irreversible cardiac damage does occur. Recent meta-analyses have shown cardiac dysfunction and HF to be a relatively common problem with TKIs, but the true incidence of TKI-induced cardiomyopathy with each TKI, and what the ideal screening and monitoring period is for these patients, has yet to be determined.
MICROTUBULE INHIBITORS
The taxane family of chemotherapy agents includes
paclitaxel and
docetaxel derived from the
Taxus genus of plants. They exert their antineoplastic effect by disrupting the function of microtubules, which are vital for cell division. The taxanes have been used since the 1990s to treat solid organ tumors including breast, ovarian, and non-small cell lung cancers. They stabilize guanosine diphosphate-bound tubulin in the microtubules, thereby inhibiting the process of cell division, thus halting mitosis altogether.
Arrhythmias are the most common side effect with taxanes, including bradycardia and heart block, suggesting the possibility that microtubules play a role in calcium handling. It is speculated that
paclitaxel decreases the calcium amplitude and contraction of isolated cardiomyocytes, but shortens the time from maximum contracted state to relaxation. In general, bradyarrhythmias are asymptomatic and self-limited, but supraventricular tachyarrhythmias such as atrial fibrillation, atrial flutter, and atrial tachycardia have all occurred.
Docetaxel has been shown to potentiate the cardiotoxic effect of anthracycline therapy.
9 Cardiotoxicity with the vinca alkalkaloids is rather uncommon, and may be more common with
vinblastine than with
vincristine and
vinorelbine. Symptoms usually include hypertension, myocardial ischemia, infarction, and other vaso-occlusive complications.
ANTIMETABOLITES
Antimetabolites are a class of chemotherapeutics that interfere with DNA and RNA growth by substituting the building blocks of DNA/RNA and damaging the proliferating cells during the S phase of mitosis. Commonly used antimetabolite drugs include:
5-fluorouricil (5-FU), cytarabine, gemcitabine, capecitabine, methotrexate, and
hydroxyurea and are used to treat solid tumors such as breast, ovarian, and gastrointestinal.
Fluorouracil’s incidence of cardiotoxicity can approach 20%.
The pathophysiology of cardiotoxicity is multifactorial with several speculated mechanisms. Endothelial damage, thrombosis, and increased oxidative stress all lead to cellular damage; coronary artery spasm leads to myocardial ischemia and thus reduced oxygen delivery. The most notable CV side effects include myocardial ischemia, angina, and ECG changes including ST-T wave and T wave changes.
Generally, cardiotoxicity occurs within the first few doses, and is much more likely to occur with higher doses or continuous infusions. Currently there are no recommendations to pre-treat patients with anti-anginal drugs, such as nitroglycerin or calcium channel blockers. However, some small trials have shown improvement in angina symptoms with
5-FU or
capecitabine when calcium channel blockers or nitrates are used. Not surprisingly, the incidence of ischemia related to
5-FU is much higher in patients with known underlying CAD.
1 A subsequent re-challenge with
5-FU usually reproduces symptoms.
Capecitabine is a prodrug that is metabolized to its active moiety,
5-FU, and has cardiotoxicity similar to infused
5-FU. Patients that have vasospasm induced by
5-FU will likely experience recurrent symptoms with capecitabine and accordingly, management of cardiotoxicity is similar to that with
5-FU. Fludarabine can cause chest pain and hypotension, and the combination of
fludarabine with
melphalan as a conditioning regimen prior to stem cell transplantation has also been associated with cardiac dysfunction.
10
PROTEOSOME INHIBITORS
The proteasome plays a key role in the maintenance of cardiac structure and function, and the ubiquitin-proteasome system regulates protein turnover in addition to activating cell-signaling pathways for growth and survival of tumors. Bortezomib (Velcade
®), the first proteasome inhibitor available for clinical use, suppresses plasma cell proliferation and is used to treat multiple myeloma.
Currently, there are only few reports of HF occurring during treatment.
Carfilzomib, one of the newer proteasome inhibitors, is an irreversible proteasome inhibitor used for relapsed multiple myeloma. It has a much greater tendency to precipitate CV events such as acute coronary syndrome, sudden cardiac death, and HF.
TREATMENT OF CTRCD (Chemotherapy-Related Cardiac Dysfunction)
There are no formal guidelines from the American Heart Association/American College of Cardiology or Heart Failure Society of America for the treatment and management of chemotherapy/radiation-induced cardiotoxicity, but the European Society of Medical Oncology recommends serial monitoring of cardiac function and initiation of therapy with ACE inhibitors and beta-blockers in patients who develop LV dysfunction, or prophylactically in patients at high risk of cardiotoxicity.
11
These recommendations are based on several small clinical studies. Recent prospective studies have looked at the cardioprotective role of ACE inhibitors and beta-blockers during chemotherapy (Fig. 2).
Fig. 2.
The Cardioprotective Role of ACE Inhibitors and Beta-Blockers During Chemotherapy
Extensively adapted from Plana JC, Galderski M, Barac A, et al. Expert Consensus for Multimodality Imaging Evaluation of Adult Patients during and after Cancer Therapy: A Report from the American Society of Echocardiography and the European Association of Cardiovascular Imaging. J Am Soc Echocardiogr 2014; 27: 911-939.
Early detection of cardiotoxicity provides an opportunity to prevent or reverse progression to advanced and end-stage HF, but there is still a paucity of data to guide selection of surveillance intervals, imaging technologies, or biomarker tests, nor what thresholds should trigger initiation of pharmacotherapy to prevent further toxicity.
A key study showed that a critical factor in cardiac recovery from anthracycline-induced cardiac dysfunction is the elapsed time from the end of chemotherapy to the start of HF therapy with ACE inhibitors and beta-blockers.
6
The PRADA (Prevention of Cardiac Dysfunction During Adjuvant Breast Cancer Therapy) trial was a randomized, placebo-controlled, double-blind study to determine whether an angiotensin receptor blocker
(candesartan), and/or a beta-blocker
(metoprolol) may prevent the development of LV dysfunction in patients receiving standard chemotherapy for early breast cancer.
12 A total of 120 patients were randomized: LV ejection fraction declined 0.8% in the
candesartan group, versus 2.6% in the placebo group (P=0.026). The authors concluded that
candesartan protected against a decline in LVEF, whereas metoprolol did not. Many argued that this randomized study should have used
carvedilol instead of
metoprolol, but until recently all studies in the cardio-oncology literature had been retrospective in design and observational.
In another prospective trial (OVERCOME), 90 patients with acute leukemia undergoing anthracycline chemotherapy were randomized to start
enalapril and
carvedilol versus placebo.
13 At six months, prophylactic
enalapril and
carvedilol stabilized LVEF compared with placebo, but the difference of 3.1 by echocardiography did not reach statistical significance (P=0.09).
In the MANTICORE study, which recently reported five-year results, the ACE inhibitor
perindopril and the beta-blocker
bisoprolol were evaluated in patients with HER2-positive early breast cancer who received
trastuzumab.
14 The combination therapy protected against declines in LVEF, with fewer interruptions in therapy. Secondary analyses of 33 patients who received
perindopril, 31 patients who received
bisoprolol, and 30 who received placebo for one year, along with
trastuzumab, showed that
bisoprolol was more effective than
perindopril or placebo in preventing chemotherapy-mediated decline in LVEF: (–1% ± 5%) vs. (–3% ± 4%) and (–5% ± 5%) respectively (P = .001).
However, cardiac MRI after 17 cycles of
trastuzumab showed that indexed LVEDP (left ventricular end diastolic volume), increased in patients treated with
perindopril (+7 ± 14 mL/m
2),
bisoprolol (+8 mL ± 9 mL/m
2), and placebo (+4 ± 11 mL/m2; P = .36). Thus, neither therapy prevented
trastuzumab-mediated LV remodeling, as reflected in LVEDP as a surrogate for LV remodeling. The study was stopped early because this primary end point was not met, but the authors, and experts in the field, did conclude that identification of CV risk factors and optimization prior to initiation of cancer therapy is a key prevention strategy for those at highest risk of developing cardiotoxicity.
It is anticipated that larger randomized clinical trials will help further delineate which HF therapies provide the greatest long-term benefits. In this regard, those active in the field of cardio-oncology strive to keep pace with the rapid evolution of chemotherapeutics, and the incidence and consequences of treatment-related CV side effects.
RADIATION-INDUCED HEART DISEASE
Patients who receive radiation therapy are also at risk for long term CV toxicity. Radiation-induced heart disease (RIHD) usually manifests 10-15 years post exposure and can contribute to the development of: restrictive cardiomyopathy, valvular and pericardial disease, premature coronary artery disease, systolic and diastolic dysfunction, dysrhythmias, autonomic dysfunction, and peripheral artery disease. Long term risk increases dramatically in patients younger than 50 years of age; when the cumulative dose of radiation exceeds 30 Gray (Gy) or the fractional doses exceed 2 Gy per day; with radiation delivered to the anterior chest or left chest; with lack of adequate shielding; when the tumor is near the heart; and if concomitant antineoplastic agents are given, especially anthracyclines.
15
Long-term follow-up and evaluation should include a yearly physical examination with a focused assessment for signs and symptoms of peripheral vascular disease and RIHD. Asymptomatic patients should receive a transthoracic echocardiogram five years post exposure in high risk individuals and 10 years post exposure in all other patients. Similarly, a cardiac stress test should be performed five years post exposure in high risk patients and after 10 years in all others to assess for accelerated CAD secondary to radiation vasculopathy.
15 Concurrent metabolic risk factors such as hypertension, tobacco use, obesity, and diabetes, increase the incidence and severity of cardiac disease.
Early reports indicated that the proximity of the heart to the chest wall in breast cancer and Hodgkin’s lymphoma increased the occurrence of RIHD, and there were higher morbidity and mortality rates in patients with left-sided breast cancer compared with right-sided cancer. However, later reports from the 1993 Surveillance, Epidemiology, and End Results (SEER) database demonstrated that there was no difference in cardiac morbidity between left- and right-sided breast cancer treated with radiation therapy.
16 After 1979, regardless of which breast, adding radiation to anthracycline treatment increased the risk of valvular heart disease and HF, but not the risk of myocardial infarction. This reduction in infarction risk was attributed to a change in radiation technique and delivery.
17
The pathophysiology of RIHD is still not thoroughly understood, but it is thought to be related to fibrosis within the myocardium, pericardium, valves, and blood vessels. The cusps and leaflets of the heart valves undergo fibrotic changes with and without calcification, and for reasons still not understood, left-sided valves are more commonly affected by radiation – which suggests that higher systemic pressures may play a role. Recent studies show a decline in RIHD in the past few decades, doubtless due to changes in delivery techniques, use of linear accelerators, decreases in variation of doses at field edges, and use of computed tomography simulation to help exclude the cardiac silhouette from tangential beams.
1 Nevertheless, we continue to treat patients that were given radiotherapy during various time periods, especially childhood cancer survivors now in late adulthood, and we must be aware of the spectrum of vascular, myo-pericardial and valvular heart disease, and aggressively treat modifiable risk factors.
ASSESSMENT OF LEFT VENTRICULAR FUNCTION
The European Society of Medical Oncology has published algorithms for serial cardiac monitoring of patients at risk for type I and type II CTRCD. In 2014 the American Society of Echocardiography with the European Association of Cardiovascular Imaging published a consensus statement on imaging assessment of patients during and after cancer treatment.
11,18
All algorithms begin with a baseline assessment of LV function and use of echocardiography as the modality of choice for serial assessment because of its wide spread availability and lack of radiation exposure. Radionucleotide angiography and cardiac MRI can also be used, but the same modality should be utilized for all follow-up imaging. If initial assessment shows an abnormal LVEF, Cardio-oncology consultation should be sought for recommendations and possibly pharmacotherapy prior to initiation of chemo- or radio-therapy.
If the baseline LVEF is normal, then timing and follow-up of subsequent EF assessment is based on whether the cardiotoxic agent is associated with type I or type II CTRCD. (Fig. 2) If LV dysfunction is detected during chemotherapy, referral to a cardio-oncologist should be made promptly for immediate initiation of ACEi/ARB and/or beta-blocker.
Time to initiation of therapy has been shown to be clinically relevant in patients receiving type I cardiotoxic agents. In patients experiencing type II CTRCD, the offending agent can usually be reinitiated after a period of discontinuing the cardiotoxic drug. Whether use of medical therapy such as beta-blockers and/or ACE inhibitors/ARBs offer clinically significant improvements in outcomes in Type II cardiotoxicity is still unknown; experts argue that given the mechanism of toxicity caused by type II agents, simply discontinuing the agent temporarily may be sufficient to allow full myocardial recovery since there is no irreversible damage to cardiomyocytes. Until unequivocal, evidence-based, clinical guidelines are available, our practice is to treat all patients with reduced EF with HF therapies regardless of type I or II CTRCD and to optimize medications to allow minimal interruptions to chemotherapy.
When monitoring for CTRCD, one significant limitation of echocardiography is that changes in EF secondary to chemotherapy often occur when irreversible damage to cardiomyocytes has already occurred. Since early initiation of medical therapy increases the likelihood of subsequent improvement in EF, determining the best methods to detect cardiotoxicity before it manifests as a decline in LVEF is an area of intense and ongoing research.
One technique that has shown promise is left ventricular strain and strain rate imaging. Global longitudinal strain (GLS) can be incorporated into routine echocardiography with readily available technology. For predicting declines in LVEF, subclinical LV dysfunction, or acute heart failure, several reviews have found GLS to have sensitivity ranging from 65-86% and specificity from 73-95%.
19
An Expert Consensus Panel concluded from the available data that a reduction in GLS between studies of less than 8% is not clinically relevant, a reduction greater than 15% is a harbinger of subclinical LV dysfunction, and a reduction of 8-15% is a gray zone that warrants closer follow-up.
18 At Lancaster General Health, we use the Phillips iE33 (Phillips Medical System) ultrasound machine to perform two- and three- dimensional echocardiography and LV GLS. Table 2 lists the normal values by gender and age (divided into deciles), and as can be seen, they can vary substantially.
20
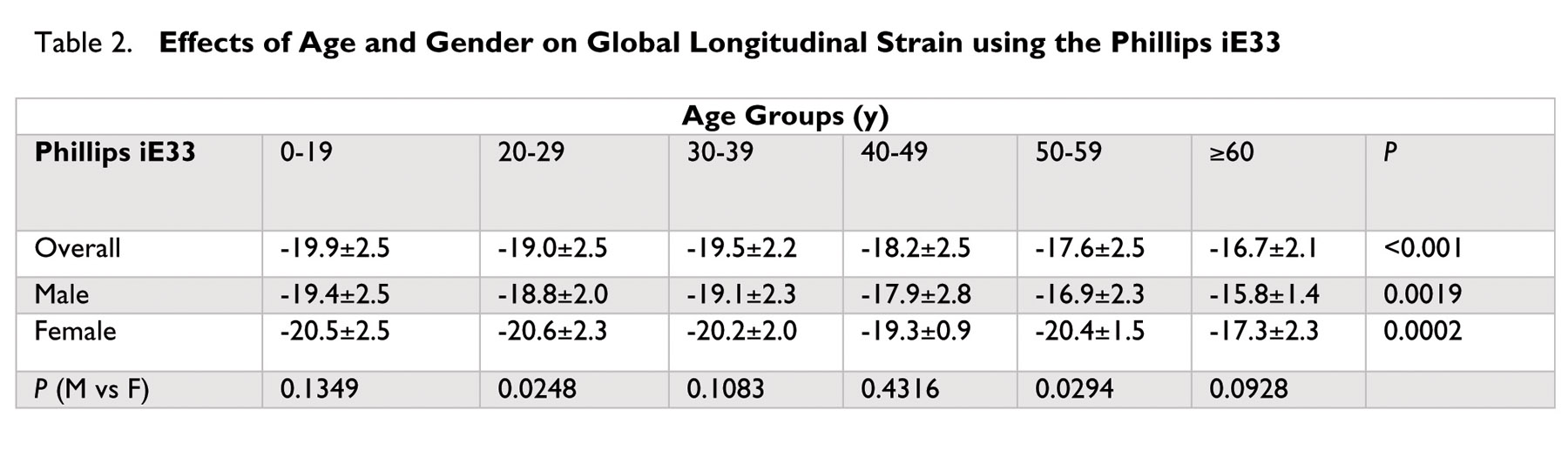
As noted earlier, it is not certain whether treatment when subclinical LV dysfunction is first identified will prevent clinically significant HF or a loss of LV function; current data do not support treating subclinical LV dysfunction. The Expert Consensus Panel advises a repeat echocardiogram within two to three weeks when a significant change in LVEF is noted on echocardiography. They also caution that when making major clinical decisions such as discontinuing potentially life-saving chemotherapy, it may be sensible to obtain cardiac MRI, either to confirm a significant drop in LVEF, or if estimation of LV function by echocardiogram is unreliable or difficult to ascertain.
18
BIOMARKERS
Cardiac troponins
Cardiac troponin I (cTnI) is the gold standard biomarker when diagnosing myocardial injury in chemotherapy patients, and is both sensitive and specific; in patients treated with anthracyclines, elevation in cTnI identifies patients at risk for the development of subsequent CTRCD.
21
One large study of 703 patients measured cTnI levels with each cycle of high dose anthracycline chemotherapy, and one month after completion.
6 The majority of events occurred in cTnI positive patients, and a persistent increase in troponin was associated with an increase in the severity of CTRCD and a higher incidence of cardiac events compared with only a transient increase. Troponin had a 99% negative predictive value, in that patients who had continually negative troponin determinations remained at the lowest risk for CTRCD. A number of smaller studies have also demonstrated a correlation between troponin levels and LV dysfunction.
22
Troponin may also be used to identify early cardiac injury in patients receiving treatment with HER2 antagonists. The largest study conducted evaluated 251 patients receiving
trastuzumab and found that cTnI positivity was associated with an increased incidence of cardiac events and lower likelihood of cardiac recovery.
In recent studies, up to 11% of patients receiving TKIs and VEGF inhibitors had elevated cTnI levels, but beta-blockers and aspirin normalized them, which translated into no increases in cardiac events during follow-up.
It remains unclear whether optimal timing of troponin assessment, or a single assessment, have prognostic implications. Currently, we do know that patients with negative troponins during chemotherapy (checked post cycle), have a lower risk of cardiac events or a drop in LV function than those with a positive cTnI.
1 Patients with a positive cTnI while receiving chemotherapy should be referred to cardio-oncology for intense medical optimization, further risk stratification, and close follow-up and monitoring for LV dysfunction.
NT-proBNP
N-terminal prohormone of brain natriuretic peptide (NT-proBNP) is another biomarker that has recently been evaluated for detection of early LV failure in chemotherapy patients. Though studies are ongoing, we still lack data to support its routine use. However, since acute elevations of NT-proBNP levels raise concern for increased LV filling pressures, its negative predictive value may be more useful, even though its variability in levels over time limits its utility in heralding a decline in LV function.
CONCLUSIONS
In the 21st century, as medicine has become more subspecialized and – as a result – increasingly fragmented, it has become increasingly important to have close collaboration between the medical subspecialties. The rapidly emerging discipline of Cardio-oncology takes a multidisciplinary approach, with alliances among cardiologists, oncologists, and hematologists. All work together for prevention, early detection, and management of CV diseases in cancer patients throughout all stages of treatment and continuing into survivorship.
As newer antineoplastic agents are approved, the magnitude of long-term CV morbidity is likely to increase, with unknown long-term sequelae. Ideally, a close partnership and open discussion of patient management options between oncologists and cardiologists will ensure optimal care for patients receiving cardiotoxic therapies.
* pôrt manto – noun – a word blending the sounds and combining the meanings of two others, for example motel (from “motor” and “hotel”) or brunch (from “breakfast” and “lunch”).
REFERENCES
1. Lenneman CG, Sawyer DB. Cardio-Oncology: An update on Cardiotoxicity of Cancer-Related Treatment. Circ Res 2006;118: 1008-1020.
2. Lenneman CG, Abdallah WM, Smith HM, et al. Sympathetic nervous system alterations with HER2+ antagonism: an early marker of cardiac dysfunction with breast cancer treatment? E Cancer Medical Science 2014; 8: 446.
3. Geisberg CA, Sawyer DB. Mechanisms of of anthracycline cardiotoxicity and strategies to decrease cardiac damage. Curr Hypertens Rep. 2010; 12: 404-410.
4. Shan K, Lincoff AM, Young JH. Anthracycline-induced cardiotoxicity. Ann Intern Med 1996;125: 47-58.
5. Cardinale D, Colombo A, Torrisi R, et al. Trastuzumab-induced cardiotoxicity: clinical and prognostic implication of troponin I evaluation. J Clin Oncol 2010;28: 3910-3916.
6. Cardinale D, Sandri MT, Colombo A, et al. Prognostic value of troponin I in cardiac risk stratification of cancer patients undergoing high dose chemotherapy. Circulation 2004;109: 2749-2754.
7. Smith LA, Cornelius VR, Plummer CJ, et al. Cardiotoxicity of anthracycline agents for the treatment of cancer: systematic review and meta-analysis of randomised controlled trials. BMC Cancer 2010;10: 337.
8. Moja L, Tagliabue L, Balduzzi S, et al. Trastuzumab containing regimens for early breast cancer. Cochrane Datab Syst Rev 2012; 4: Cd006243.
9. Malhotra V, Dorr VJ, Lyss AP, et al. Neoadjuvant and adjuvant chemotherapy with doxorubicin and docetaxel in locally advanced breast cancer. Clin Breast Cancer 2004;5 : 377-384.
10. Van Besien K, Devine S, Wickrema A, et al. Regimen related toxicity after fludarabine-melphalan conditioning: a prospective study of 31 patients with hematologic malignancies. Bone Marrow Transplant 2003;32 : 471-476.
11. Bovelli D, Platanitotis D, Roila F. ESMO Guidelines Working Group. Cardiotoxicity of chemotherapeutic and radiotherapy-related heart disease: ESMO Clinical Practice Guidelines. Ann Oncol 2010;21(Suppl5): v277-v282.
12. Heck SL, Gulati G, Ree AH, et al. Rationale and design of the prevention of cardiac dysfunction during an adjuvant breast cancer therapy (PRADA) trial. Cardiology 2012; 123: 240-247.
13. Bosch X, Rovira M, Sitges M, et al. Enalapril and carvedilol for preventing chemotherapy-induced left ventricular systolic dysfunction in patients with malignant hemopathies: the OVERCOME trial. J Am Coll Cardiol 2013; 61: 2355-2362.
14. Pituskin E, Mackey JR, Koshman S, et al. Multidisciplinary Approach to Novel Therapies in Cardio-Oncology Research (MANTICORE 101-BREAST): A Randomized Trial for the Prevention of Trastuzumab-Associated Cardiotoxicity. J Clin Oncol 2017; 35 : 870-877.
15. Lancellotti P, Nkomo VT, Badano LP, J, et al. 2013 Expert consensus for multi-modality imaging evaluation of cardiovascular complications of radiotherapy in adults: a report from the European Association of Cardiovascular Imaging and the American Society of Echocardiography. J Am Soc Echocardiogr 2013; 26: 1013-1032.
16. Patt DA, Goodwin JS, Kuo YF, et al. Cardiac morbidity of adjuvant radiotherapy for breast cancer. J Clin Oncol 2005; 23: 7475-7482.
17. Hooning MJ, Botma A, Aleman BM, et al. Long-term risk of cardiovascular disease in 10-year survivors of breast cancer. J Natl Cancer Inst 2007; 99: 365-375.
18. Plana JC, Galderski M, Barac A, et al. Expert Consensus for Multimodality Imaging Evaluation of Adult Patients during and after Cancer Therapy: A Report from the American Society of Echocardiography and the European Association of Cardiovascular Imaging. J Am Soc Echocardiogr 2014; 27: 911-939.
19. Thavendiranathan P, Poulin F, Lim KD, et al. Use of myocardial strain imaging by echocardiography for the early detection of cardiotoxicity in patients during and after cancer chemotherapy: a systematic review. J Am Coll Cardiol 2014; 63: 2751-2768.
20. Takigiku K, Takeuchi M, Izumi C, et al. Normal range of left ventricular 2-dimensional strain: Japanese Ultrasound Speckle Tracking of the Left Ventricle (JUSTICE) study. Circ J. 2012; 76: 2623-2632.
21. Lenihan DJ, Massey MR, Baysinger KB, Adorno CL, Warneke CL, et al. Superior detection of cardiotoxicity during chemotherapy using biomarkers. J Card Fail. 2007; 13 : S151.
22. Ky B, Putt M, Sawaya H, et al. Early increases in multiple biomarkers predict subsequent cardiotoxicity in patients with breast cancer treted with doxorubicin, taxanes, and trastuzumab. J Am Coll Cardiol 2014; 63: 809-816.