ABSTRACT
Despite the availability of a wide variety of foods and supplements, and a growing interest in nutrition, the incidence of Vitamin D deficiency is on the rise in the United States. Because vitamin D enhances the availability of calcium for bone mineralization, disorders marked by a loss of bone strength are likewise becoming more common. The causes of this trend are myriad—from inadequate supplementation, sun, and exercise, to excessive smog, clothing, and medications. Diet plays a relatively minor role in vitamin D deficiency because of the limited number of foods that contain substantial amounts. Aging is more important because of the loss of hormonal activity that normally inhibits bone resorption. Although laboratory tests can detect vitamin D metabolite levels with great accuracy, we unfortunately don’t know what serum levels indicate vitamin D insufficiency, so we lack the earliest warning of the need for intervention to maintain bone health. Nonetheless, because our understanding of the mechanism of action of this vitamin has advanced over the years, the recommendations for an adequate intake of vitamin D have changed, along with recommendations for supplementation with calcium. Research must continue, however, to identify the earliest point along the continuum of serum vitamin D levels that justifies therapeutic intervention.
(Note: 4 Tables and 3 Figures are posted at www.jlgh.org. See end of article for details.)
INTRODUCTION
The relationship between vitamin D and bone health, particularly as a cure for rickets, has been well understood for at least half a century. Rickets occurs in childhood prior to fusion of the epiphyses. When the epiphyses fail to mineralize because of inadequate vitamin D, they become thickened and irregular, and growth is retarded. By 1940 researchers had purified both forms of the vitamin (vitamin D2 and vitamin D3), had unraveled much of the mechanism by which it is transformed into its active form, and understood its potent effect on rickets. Also by 1940, milk manufacturers in the United States were fortifying their product with vitamin D as a public health measure against rickets, and the medical world watched this disorder spiral downward from a major disabler of young children to a subject worthy of little more than a few lines in history books. Yet sixty years later, in spite of the extension of vitamin D fortification to other foods, and the widespread awareness and availability of nutritional supplements, rickets is on the rise again.1 No one seems to be getting enough vitamin D today, and studies have shown that approximately one third of otherwise healthy young adults (18-29 y) in the United States have a low vitamin D level (<20 ng/mL).2
What happened? An increasingly sedentary lifestyle hasn’t helped, nor has inconsistent and often out-of-date information about vitamin D requirements. Neither have smog and sunscreen, both of which weaken the sun’s ability to produce vitamin D. But regardless of the causes, it is up to health care professionals to do everything possible to reverse the trend toward rising hypovitaminosis D. To do so, we must first accept that this increasingly common vitamin deficiency really does have a significant effect on the health status of our patients; we must learn how to identify the individuals at greatest risk for this disorder; we must learn how to select the most accurate method of making a diagnosis; and we must understand how to incorporate nutritional approaches into the treatment for any underlying cause of hypovitaminosis D.
This article will describe the sources, metabolism, and effects of vitamin D, and demonstrate how it can be used to limit the risk of debilitating disorders in highly vulnerable populations. It will focus on age-related changes in vitamin D status that influence bone health, in order to understand how to prevent or slow the development of bone-related disorders that accompany aging, such as osteoporosis and fractures.
A. WHAT IS VITAMIN D?
Though vitamin D is described as a fat-soluble vitamin produced by both plants and animals, it is actually a hormone that influences the activity of a variety of tissues and organs in response to changes in the amount of metabolic byproducts in the blood. Unlike conventional vitamins, most of which serve as enzyme cofactors to help catalyze intracellular biochemical reactions, vitamin D interacts with vitamin D receptors (VDRs) on the surface of cells to induce the intracellular production of specific proteins that carry out specific vitamin-D–determined effects.2,3
Vitamin D exists in several forms, of which the calciferols are the most active.1 The term “vitamin D” is used collectively to identify two molecules: vitamin D2 (ergocalciferol), which is derived from plants and used to fortify foods; and vitamin D3 (cholecalciferol), which is found in fish oils, eggs, cod liver oil, and animal fats. Another important source of vitamin D3 is the skin, where it is formed from provitamin D3 (7-dehydrocholesterol) in the plasma membrane of keratinocytes. When exposed to ultraviolet radiation (UV-B; wavelength: 290-315 nm),2,3 provitamin D3 undergoes photolysis and is converted to previtamin D3. The temperature of the skin then triggers the conversion of previtamin D3 to vitamin D3.
Metabolism of Vitamin D
Both cutaneous and ingested vitamin D are transported in the bloodstream mainly by a vitamin D-Binding Protein (DBP) which is synthesized in the liver, and to a much lesser extent by albumin. Vitamin D is converted into its physiologically active form—1,25 dihydroxyvitamin D (1,25[OH]D)—through 2 hydroxylation reactions.1,3 The first takes place in the liver, where vitamin D undergoes 25-hydroxylation, a reaction that is catalyzed by cytochrome P450-like enzymes in the hepatic mitochondria and microsomes.3 This reaction produces the vitamin D metabolite 25-hydroxyvitamin D (25[OH]D), the main form of vitamin D circulating in the blood and stored in body tissues. The half-life for this metabolite is relative long - approximately 2 to 3 weeks in healthy adults - and this hydroxylation step is relatively unregulated. Thus, serum 25(OH)D levels closely reflect substrate availability, making it the metabolite of choice for evaluating vitamin D status.4
The second hydroxylation reaction takes place in the kidney’s proximal tubule cells, where 25(OH)D is transformed into the biologically active molecule, 1,25(OH)2D in a reaction catalyzed by 25(OH)D-1-alpha-hydroxylase, a mixed-function microsomal oxidase. (*Fig. 1). The polar metabolites of this reaction are secreted into bile and are reabsorbed through the enterohepatic circulation. Two negative feedback loops appear to regulate this reaction in the kidney, as it is repressed by both 1,25(OH)2D, and by calcium. The absorption of calcium is enhanced by vitamin D and by parathyroid hormone (PTH), which increases calcium levels in the blood by promoting its release from bone (*Fig. 2).2
Mechanism of Action
The primary bone-related function of vitamin D is to maintain calcium homeostasis. Vitamin D activity begins when 1,25(OH)2D binds with a vitamin D receptor (VDR).3 This receptor, which is expressed in a variety of tissues, mediates the regulation of gene expression by forming a complex with those DNA sequences that express proteins which influence gene expression.2 The VDR/DNA complex can induce the expression of proteins that participate in bone formation, such as calbindin 9K, an intestinal calcium-binding protein that is believed to facilitate the active transport of calcium across the gut wall; the bone matrix proteins osteocalcin and osteopontin, which are secreted by osteoblasts and help calcium bind to the bone matrix during the bone mineralization process; and type I collagen, which is also secreted by osteoblaststo form the primary component of the bone matrix and undergo calcification to form mature bone. VDR also suppresses the transcription of genes that express parathyroid hormone (PTH), and since PTH promotes the mobilization of calcium from bone, VDR thus inhibits resorption. However, VDR can also induce up-regulation of the RANK ligand, which promotes the differentiation and activity of osteoclasts, which participate in resorption (*Fig. 2). By balancing the expression of gene products that favor bone production with those favoring bone resorption, vitamin D allows bone to adapt rapidly to changes in load and tension while maintaining bone strength.
Vitamin D sources
Sunlight’s importance as the primary source of vitamin D varies in different populations depending on latitude and the availability of oral supplementation.2 Although sunlight is most effective between latitudes 37° north and 37° south,2 it has been known to provide as much as 85% of the vitamin D intake in elderly individuals as far as 57° north latitude.5 Food sources of vitamin D in the United States are limited mainly to fortified foods, especially orange juice, cereals, and, of course, milk (interestingly, many milk products—such as yogurt, cheese, and ice cream—are usually not fortified). The best nonfortified food sources are cod liver oil and oily fish, such as salmon, mackerel, and sardines (Table 1*).
Both of the most common forms of vitamin D—vitamin D2 (ergocalciferol) and vitamin D3 (cholecalciferol)—are available as dietary supplements. Vitamin D3 is preferred because of its superior bioactivity.
B. ROLE OF VITAMIN D IN PROMOTING BONE STRENGTH
Bone strength is promoted by the incorporation of calcium into the matrix of bone, which consists primarily of type I collagen. The rigidity of bone increases with the density of its mineral content, while the flexibility of bone (ie, its ability to resist tension) depends on its mineral content as well as the number of collagen cross-linkages, which keep the triple-helical collagen structures anchored to each other.6
Vitamin D contributes to bone strength primarily by facilitating the absorption of calcium from the small intestine and inhibiting PTH activity to prevent the loss of calcium from bone.2 (When 25(OH)D levels fall, less calcium is available to be incorporated into the bone matrix. Low plasma calcium levels trigger an increase in the production and release of PTH, which, in turn, induces the mobilization of calcium from bone to restore serum calcium levels and increase the production of 1,25[OH]2D).
C. BONE: A REVIEW
1. Function and Structure of bone
For bones, function determines structure. The two types, cancellous and compact, are arranged and proportioned in bones according to their function as a lever (long bones) or a spring (vertebral bodies). Even within the same bone, the distribution of cancellous and cortical bone is determined by the work load of different sections. The femoral neck, for example, is circular and highly trabecular close to the femoral head to withstand compressive stress, but elliptical and thick at the end of the shaft to minimize bending.
Cortical bone is composed of bony columns that lie parallel to the bone’s long axis, and consist of concentric layers of osteocytes, which are actually osteoblasts that became trapped in the bone matrix and “lost” their ability to build bone. Instead, they mediate the release or deposit of calcium in bone in response to calcium, PTH, and calcitonin levels in the blood. Each column surrounds a hollow canal (the Haversian canal) containing neurovascular bundles that connect with each other and with the endosteum (inner layer) and periosteum (outer layer) of bone. The periosteum and endosteum are both lined with tissue containing osteoprogenitor cells (the predecessors of osteoblasts), which participate in bone remodeling and repair. The endosteal lining also contains osteoblasts and osteoclasts, which participate in bone remodeling.6
Cancellous bone (also known as trabecular bone) is thin and irregular and forms a labyrinth of spaces which contain bone marrow. Its structure confers a light, flexible character to bone, thereby facilitating the absorption of a considerable amount of energy, but at the expense of being unable to bear as much of a load as cortical bone.
2. Bone Remodeling
Remodeling in response to rapid changes in mechanical stress and serum calcium levels is mostly carried out by osteoblasts and osteoclasts primarily in the endosteum, and to a lesser degree, in the periosteum. Osteoblasts synthesize and secrete osteoid, the organic matrix of bone that undergoes rapid mineralization to form a new, functional bone. Osteoclasts are multinucleated cells that participate in bone resorption. It is crucial for bone resorption and deposition to occur at approximately the same rate to prevent a loss of bone strength. Both rates decline with age, a phenomenon that is most apparent beginning in midlife, but may begin during early adulthood (age 18-30 y).
Resorption usually begins when osteocytes detect damaged or deformed bone, then send signals to regulate the amount of remodeling suitable for the change in load or pressure. We suspect that osteocytes in the damaged tissue undergo apoptosis, which triggers the release of biochemical and chemotactic signals that are detected by osteoclasts, which actually remove the damaged tissue. Osteoclastogenesis is mediated by vascular growth factors, osteoclast precursors, osteoblast precursors, macrophages, and activated T cells. Osteoclasts are formed from precursors that are released into the bloodstream as monocytes, fuse to form multinucleated cells, and aggregate at bone resorption sites.
a. The remodeling process
Multinucleated osteoclasts tunnel into existing bone, forming cavities that will be invaded by capillaries. Osteoblasts then line the capillary walls and secrete osteiod to lay down new concentric layers of bone matrix that gradually fill the tunnel, leaving only a narrow canal surrounding the capillary. Many osteoblasts will become trapped in the bone matrix, where they are transformed into osteocytes and organize themselves into concentric rings. Simultaneously, osteoclasts break down other concentric systems elsewhere in the bone.7
b. Factors influencing bone remodeling rates
Type of bone. Trabecular bone contains more remodeling sites than cortical bone because it has a greater surface area, so there is a greater turnover of trabecular bone. The combination of a high remodeling rate and the formation of resorption cavities that are deep in relation to the thinness of the trabeculae, results in a greater loss of strength in trabecular bone than in cortical bone in response to metabolic changes. Women are more susceptible to these changes than men, because of the loss of estrogen with menopause, coupled with the reduced volume of bone seen with aging. The greater vascularity of trabecular bone results in a more rapid loss of bone density in the spine than in the hip in postmenopausal women.6
Sex hormones. Estrogen stimulates osteoblasts and inhibits PTH-activated osteoclasts.8 Androgen also affects bone remodeling, both directly - through androgen receptors on osteoblasts, osteoclasts, and osteocytes - and indirectly through its effect on growth factors (eg, insulin growth factor-I) that influence the rate of bone growth.9 Declines in gonadal function in both men and women, as well as aging in general, reduce these effects, resulting in secondary hyperparathyroidism and increased rates of remodeling. The end result is an increase in the formation of trabeculae in cortical bone. The resulting increase in surface area increases the risk of bone perforation, and reduces the amount of energy the bone can absorb, eg, during a fall.6
D. ASSESSMENT OF VITAMIN D STATUS
A patient’s nutritional status for any nutrient is usually determined by measuring its serum level or the level of a biomarker for that nutrient (in the case of vitamin D - 25[OH]D), and comparing it with expected levels. For vitamin D, this approach is hampered by two important factors: (1) serum test results can vary remarkably among laboratories, and (2) the minimal effective serum vitamin D level has not been agreed upon.
Fortunately, methods are available to guide the evaluation of a patient’s status well enough to make an accurate diagnosis and develop an appropriate treatment plan.
1. Measuring 25(OH)D Levels
Measurement of 25(OH)D levels is complicated, because it is predominantly bound to DBP (approx 85%) and albumin (15%) in the serum; it must be extracted from these proteins before it can be measured accurately using chromatographic techniques. Another problem is the fact that its serum levels are extremely low (in the nanomolar range).4 The most common assays are the Nickels Advantage assay (a chemiluminescence protein-binding assay) and the DiaSorin radioimmunoassay, but the benchmark assays are high-performance liquid chromatography (HPLC) and LC-mass spectrometry (LC-MS) assays.4 (The LC-MS assay was introduced to the Lancaster General Hospital laboratory in September 2006.).
In a recent study by the Vitamin D Quality Assessment Scheme (DEQAS), there was a considerable amount of interlaboratory variability in 25(OH)D measurements. Most of the test results fell within acceptable limits, but the automated Nichols Advantage assay appeared to underestimate 25(OH)D2 levels, and the assay’s manufacturer added a warning about underrecovery to the assay kit.10 Because there is some inter-test variability, it is important to use the same laboratory to test patients whose 25(OH)D levels are being monitored to assess their response to treatment.
2. The 25(OH)D Continuum Controversy: A Question of “Inadequacy”
Circulating 25(OH)D levels are considered “normal” if they can keep PTH levels within normal limits; values exceeding 30 ng/mL (75 nmol/L) are generally considered adequate for this purpose. When they fall below 30 ng/mL, PTH levels start to rise, and they keep doing so if the 25(OH)D level continues falling. If 25(OH)D falls below 10 ng/mL (25 nmol/L), the PTH level usually spikes, giving clear evidence of a vitamin deficiency.
Our current need is to identify the 25(OH)D level at which PTH activity first becomes critical, since that level could be used to indicate vitamin D insufficiency, i.e. the point at which patients should be advised to start taking a supplement, increasing sun exposure, and planning their diet to maximize vitamin D intake. We expect that level to lie between 10 and 30 ng/mL (25-75 nmol/L), but though our understanding of the effects of various points along this continuum is expanding gradually, the exact inflection point remains to be determined (*Fig. 3).
We do know that the efficiency of calcium absorption increases up to 25(OH)D values of approximately 32 ng/mL (80 nmol/L), then slows down considerably; we know that the risk of osteoporotic fractures is lower at 30 ng/mL (75 nmol/L) than at 20 ng/mL; we know that the bone mineral density (BMD) in the hip and neuromuscular activity in the lower extremities increase dramatically at levels of 16 to 20 ng/mL (40 to 50 nmol/L); and we know they continue to improve (albeit at a slower pace) up to 40 ng/mL (100 nmol/L). It has also been estimated that an individual with a level of about 20 ng/mL (50 nmol/L) would need to take 1000 to 2000 IU of vitamin D every day to reach the “acceptable” level of 32 ng/mL (80 nmol/L). Because 25(OH)D levels lower than 10 ng/mL (25 nmol/L) indicate vitamin D insufficiency with reasonable certainty, physicians know that an aggressive treatment plan is probably appropriate for patients at that point along the continuum.11 Table 2* summarizes physiological changes that have been reported in the literature for specific serum 25(OH)D levels.
3. Vitamin D Inadequacy
Despite the wide availability of fortified foods and supplements, and moderately good access to sunlight, a broad spectrum of the U.S. population has inadequate serum levels of vitamin D. Hospitalized patients are particularly vulnerable. As many as 57% of general medical patients in the United States have 25(OH)D levels <20 ng/mL, and in Europe the incidence is even higher (70%-100%). Postmenopausal women, especially those with osteoporosis and a history of fracture, are also more likely to have vitamin D inadequacy.3 In those being treated for osteoporosis, 36% have vitamin D levels below 25 mg/mL and 18% have levels below 20 mg/mL.12
In addition to inadequate exposure to sunlight and certain metabolic disorders, eight major demographic factors have been identified: advanced age (>80 y), race (nonwhite),1 obesity (BMI >30 kg/m2), concomitant use of medications that interfere with vitamin D production, inadequate vitamin D supplementation (<400 IU/d), sedentary lifestyle, lack of communication with a physician regarding the role of vitamin D in bone health, and limited education (<12th grade). Strict vegetarianism--especially with a diet that does not include milk and milk products, fish, or both—may also contribute.1,2,12
a. Inadequate exposure to sunlight
The cutaneous production of vitamin D can be compromised by such basic factors as geography, clothing, and clouds. At latitudes above 37° north or below 37° south, the sun doesn’t shine long enough during the winter months to induce adequate cutaneous vitamin D3 production. Women who keep most of their bodies covered throughout the day for cultural and/or religious reasons (e.g., devout Muslim women) have a high incidence of osteomalacia and a high rate of rickets among their children. (Osteomalacia is due to unmineralized osteoid, the same abnormality as in rickets, but it occurs in adults after fusion of the epiphyses.) A complete cloud cover (or smog) can reduce the energy in the sun’s UV rays by as much as 60%, thereby reducing cutaneous vitamin production significantly, as can increased skin pigmentation and the regular use of sunscreen with an SPF higher than 8.1
b. Metabolic risk factors
The major pathway for inactivation of vitamin D metabolites is through vitamin D-24-hydroxylase which is expressed in most tissues. Since the major inducer of vitamin D-24 hydroxylase is 1,25(OH)2 vitamin D, it promotes its own inactivation. Interestingly, metabolites of 1,25 (OH)2 vitamin D are secreted into the bile and reabsorbed via the enterohepatic circulation, so diseases of the terminal ileum can impair this circulation and lead to accelerated losses of vitamin D. Similarly, because vitamin D is a fat-soluble vitamin that is sequestered in body fat, any condition that compromises fat absorption - e.g. sprue, cystic fibrosis, or Crohns’ disease - will also compromise vitamin D stores in the body. Severe liver dysfunction has a similar effect, because it removes the hepatic enzymes that catalyze the 25-hydroxylation of vitamin D to the metabolically active form.3,4 Abnormal kidney function that interferes with the conversion of vitamin D to its active form may also result in a deficiency state.2,3
c. Demographic risk factors
Aging. In addition to declining sex hormones, other factors that compromise vitamin D status in the elderly include lactose intolerance, and increased demand for calcium due to failing bone health.
Medications that interfere with the absorption or metabolism of vitamin D include: anticonvulsants, bile acid sequestrants, cimetidine, corticosteroids, mineral oil, orlistat, and stimulant laxatives, such as Bisacodyl, Cascara, and Senna.
Race. Vitamin D levels tend to be lower in African Americans than whites, even during young adulthood. The reasons may include a higher rate of lactose intolerance, which sharply reduces the availability of vitamin D-rich dairy foods; and greater pigmentation, which reduces the UV radiation that reaches the keratinocytes by as much as 99%.1-3
Inadequate supplementation. Current US recommendations for vitamin D supplementation may be inadequate for high-risk individuals to achieve adequate circulating levels (see “Vitamin D Dosing Strategies”).12
Sedentary lifestyle. Studies have shown that weight-bearing exercises (walking, mild to moderate aerobics, and resistance exercises) are effective in maintaining bone density in the hip and lumbar spine, particularly in postmenopausal women.14 This type of exercise also correlates directly and significantly with 25(OH)D levels.9 Therefore, a lack of exercise increases the risk for bone loss as well as the need for vitamin D.12,14 A sedentary lifestyle also often means limited exposure to the sun.
Lack of communication. Older patients have traditionally been told that they need only 200 to 400 IU of vitamin D each day from a daily multiple vitamin, but recent research suggests supplementation of 1000 IU/day or more, using vitamin D3 rather than D2 to achieve the highest vitamin D levels possible.15 Calcium therapy is recommended as an adjunct to vitamin D therapy, especially in the presence of advanced age, an inadequate dietary intake, and limited sun exposure.14
The health care professional should initiate a conversation with every at-risk patient about the importance of achieving and maintaining an adequate vitamin D status. Because having a limited education also places the patient at risk for vitamin D inadequacy,12 it is important to be aware of patient education resources for individuals with a broad range of educational achievement. Several national and international organizations provide patient-oriented information on the Web or materials and resources that health care professionals can use with their patients. They include the National Osteoporosis Foundation (www.nof.org), the National Institute of Arthritis, Musculoskeletal, and Skin Diseases (www.niams.nih.gov/bone/bone_health.htm), and the International Osteoporosis Foundation (www.osteofound.org).
E. VITAMIN D DEFICIENCY AND SECONDARY OSTEOPOROSIS
1. Osteoporosis: A Review
Osteoporosis is the most common disease of bone, causing more than 1.5 million fractures annually in the United States. It affects all races, but is most common in elderly white women.17 Osteoporosis is characterized by loss of bone substance, and chronic, progressive deterioration of the bone’s microarchitecture, leading to decreased bone strength and increased risk of fracture.14 Quantitatively, it is defined as a bone mineral density (BMD) that is 2.5 standard deviations below the mean for healthy young adults.17
a. Primary versus secondary osteoporosis
Primary osteoporosis is associated with aging and the loss of gonadal function in both men and women. As a result, remodeling favors osteoclast activity over osteoblast activity, and bone resorption pits that develop during remodeling are not completely filled.9 Secondary osteoporosis occurs as a result of a chronic medical condition, long-term medication use, nutritional deficiency, and other causes.9,14,17 It tends to occur in men (approx 30%-60% of cases) slightly more frequently than in perimenopausal women (<50% of cases).14
b. Vitamin D and the risk of fracture
Osteoporotic fractures are seen most often in vertebrae, hips, and wrists. The particular risk of vertebral fractures reflects the reduction in bone mass that accompanies aging. Since some risk factors for this decline are beyond the patient’s control (age, female sex, white or Asian race, etc), it is crucial to remedy those – such as a low intake of calcium and vitamin D – that are relatively easily corrected (Table 3*).17 As indicated in Table 2*, the threat to bone strength begins when the 25(OH)D level falls below 30 ng/mL (75 nmol/L), at which point serum PTH levels begin to rise.
2. Diagnosis
Any “fragility fracture” suggests osteoporosis. Since vertebral fractures may be asymptomatic, the physician must look for other signs, such as a decrease in height (>3 cm), or a reduced distance between the lowest rib and the pelvis (<2 finger breadths). Vertebral fractures should also be suspected if the “wall-to-occiput” distance (i.e., the kyphotic posture) is abnormal. With the patient standing with back and heels against the wall, this measurement of the horizontal distance between the rear of the patient’s head and the wall is normally zero.17
A diagnosis of osteoporosis is confirmed by measuring the patient’s BMD, for which the gold standard is central dual-energy x-ray absorptiometry (DEXA). The BMD is usually measured in the hip, although the International Society for Clinical Densitometry and other organizations recommend the posteroanterior lumbar spine, femoral neck, total hip, or distal radius in the nondominant forearm. It may also be possible to make an accurate diagnosis on the basis of findings in other locations—including the trochanter, lateral lumbar spine, other parts of the forearm, the heel, or the entire body—or by combining DXA findings with those obtained using another technology (e.g., calcaneal ultrasound, peripheral DEXA, quantitative computed tomography, single-or dual-photon radionuclide absorptiometry, or magnetic resonance imaging).
BMD is measured in terms of T- or Z-scores: the T-score is used in postmenopausal women and in men who are at least 50 years old, and represents the number of standard deviations (SDs) from the mean BMD in sex-matched normal young adults. The Z-score is used in all other patients, and represents the number of SDs from the mean BMD in age-matched individuals of the same sex.
Because the risk of osteoporosis increases with age, a BMD test is recommended for all men and women older than 65 years, and repeated testing is recommended for high-risk individuals, including menopausal women, postmenopausal females younger than 65 years with additional risk factors, and anyone with a fragility fracture.14,17 Patients who are too frail, have limited mobility, or are too obese to be taken for a DXA test are often evaluated using an osteoporosis risk assessment instrument. The sensitivity of this type of instrument is low, but it is helpful for ruling out osteoporosis in women younger than 75 years who weigh more than 60 kg and have no history of a minimal-trauma fracture.17
3. Diagnosis of Secondary Osteoporosis
The National Osteoporosis Foundation (NOF) recommends evaluating any patient with a treatable cause of osteoporosis (Table 4*) with relevant blood and urine studies before initiating therapy for osteoporosis.13 The laboratory tests that are commonly ordered include a serum chemistry profile (especially for calcium, phosphorus, albumin, alkaline phosphatase, and creatinine), thyroid stimulating hormone, 25(OH)D, PTH, and - in men - total testosterone.17 Depending on the patient’s clinical history, additional tests may be appropriate, such as a serum protein electrophoresis, celiac antibody profile, and serum cortisol level.
4. Treatment Options for Osteoporosis
The NOF recommends initiation of therapy to reduce fracture risk in women with:
- BMD scores below -2.0 by Central DEXA with no risk factors.
- BMD scores below -1.5 by Central DEXA with one or more risk factors (other than being white, post menopausal and female).
- A prior vertebral or hip fracture.
For patients with secondary osteoporosis in whom work-up for a secondary cause is either negative or not indicated, treatment begins with enough calcium and vitamin D to restore 25(OH)D levels to normal. If, however, conditions are present that can induce secondary osteoporosis (Table 4*), they should first be corrected or their effects should be minimized.
Pharmacological options include antiresorptive agents, e.g., calcitonin, raloxifene, the bisphosphonates (alendronate, risedronate, or ibandronate), and estrogen, as well as anabolic agents (i.e. PTH analogs, such as teriparatide). Estrogen is no longer approved for the treatment of patients with osteoporosis; however, it may be used to prevent osteoporosis in some postmenopausal women.15
Nonnutritional, nonpharmacological approaches include weight-bearing exercises and fall-prevention strategies - physical therapy, gait aids, the prevention and/or treatment of hypotension, avoidance of medications that alter mental state or gait stability, installation of grab bars in tub/shower or next to toilet, removal of throw rugs, use of hip protectors, etc.
E. VITAMIN D DOSING STRATEGIES
Recent findings have dramatically changed recommendations for vitamin D intake, even for healthy individuals. Because of the limited number of food sources that contain vitamin D, supplements are necessary, together with sensible increases in sun exposure.
1. Current Recommendations: Are They Too Low?
The FDA, the Institute of Medicine, and the European Scientific Committee for Food have all recommended a vitamin D intake of 400 IU/day for healthy adults older than 50 years.1 Although the FDA also recommends this dose for people of all ages, it acknowledges that it may represent the minimum requirement, particularly during winter months. By any standard, it certainly is insufficient for postmenopausal women and the elderly.18
If the therapeutic target is a level of 25(OH)D above 30 ng/mL (75 nmol/L), the FDA acknowledges that most adults need 1000 IU/day, specifically of vitamin D3 (cholecalciferol) to achieve it. Although some have suggested that 700 to 800 IU/day will suffice to reach 30 ng/mL, even in the elderly and in postmenopausal women,14 there is a growing consensus that 32 ng/mL (and perhaps even as much as 48 ng/mL) may be the minimum serum level necessary for optimal vitamin D activity.19
To hedge all bets, several investigators have recommended combining the higher vitamin D intake levels (³1000 IU/d) with an adequate calcium intake,15 especially when the patient is at risk for bone loss or the diet is already low in vitamin D. For most adults, there is little concern about vitamin D toxicity, and there are no credible reports of toxicity with serum 25(OH)D levels lower than <200 ng/mL (500 nmol/L).19 An incredibly large daily intake (40,000-100,000 IU), for a very long time, is necessary to produce such toxic levels.20
2. Sources of Vitamin D
When making recommendations about vitamin D intake to patients, all three sources should be considered: food, supplements, and sunlight.
The best dietary source of vitamin D, cod liver oil, is certainly not the most popular, but at close to 400 IU per teaspoon, it is a convenient way to get the minimum recommended amount quickly and cheaply.1 Other good sources of vitamin D are listed in Table 1*. Second, both vitamin D2 and D3 are available as dietary supplements. Unfortunately, only vitamin D2 is available for high-dose prescriptions in the United States,5 so patients who require higher doses of vitamin D3 may need help complying with the need for multiple smaller doses to achieve an adequate intake. A prescription for a 1,25(OH)2D2 may be necessary for patients with chronic kidney failure to minimize the risk of secondary hyperparathyroidism.
Third, direct sunlight can provide an important portion of a patient’s vitamin D requirement. When the arms and legs are exposed for a maximum of 5 to 10 minutes between the hours of 10 am and 3 pm without sunscreen 2 to 3 times a week during the spring, summer, and fall, a total of approximately 500 to 1500 IU of vitamin D3 is produced in the skin.16 The risk for skin cancer is minimal with this practice because of the brief duration of sun exposure. Patients who express concern about the risk of skin cancer should be reminded that the only deadly tumors—melanomas—rarely develop on parts of the body that are frequently exposed to the sun. They should also be advised to use sunscreen with an SPF higher than 8 after this exposure and check their skin for signs of this condition as a part of their regular health routine, keeping in mind that early detection results in the best chance for a cure.1
3. Calcium recommendations
The NIH recommendations for calcium intake differ according to gender and age. For women, a total calcium intake of 1000 mg/day is recommended until menopause, and 1500 mg/day thereafter. For men, an intake of 1000 mg/day is recommended until they reach their 65th year, and 1500 mg/day thereafter.14 These amounts may be difficult to obtain through the diet, particularly in the elderly, who may have lactose intolerance or a waning appetite, and they are good candidates for a supplement.
Selection of a calcium supplement
Selection of a supplement should consider its rate of absorption, convenience, cost, and risk of adverse effects. The rate of absorption is usually maximal in supplements containing no more than 500 mg of elemental calcium. The two common supplements are calcium carbonate and calcium citrate.
Calcium carbonate is approximately 40% elemental calcium and provides no more than 500 mg per tablet. Since it requires the presence of gastric acid in order to be digested and absorbed, it must be taken with meals, and it has an increased risk of nephrolithiasis than is seen with calcium citrate.
Calcium citrate is approximately 21% elemental calcium and does not require gastric acid for maximum bioavailability, so it can be taken with or without food, and is the supplement of choice for patients who take gastric-acid inhibiting drugs or have a history of kidney stones. Its only drawback is its greater cost. Both supplements have similar adverse effects, which include constipation, bloating, and gas, though they are less severe with calcium citrate.
Note that calcium itself can compromise the absorption of certain drugs, including angiotension-converting enzyme inhibitors, fluoroquinolones, and levothyroxine, and may need to be used cautiously or avoided in patients taking these drugs.
F. TREATMENT OF SEVERE VITAMIN D DEFICIENCY
Severe vitamin D deficiency, usually indicated by a 25(OH)D level lower than 10 ng/mL (25 nmol/L), is much less common than vitamin D inadequacy (<30 ng/mL [75 nmol/L]), and in the United States it is seen most often in elderly, housebound individuals. Affected patients should take a 50,000 IU oral dose of vitamin D2 each week for eight weeks, after which the 25(OH)D level should be checked. If is still not within the desired range (>30-50 ng/mL [75-125 nmol/L]) for individuals being treated for a deficiency condition), a second course of treatment may be required. If the patient is prone to hypovitaminosis D, it may be necessary at this point to prescribe 50,000 IU every 2 to 4 weeks or to initiate long-term therapy with 1000 IU/day of vitamin D3 coupled with adequate sun exposure.2
SUMMARY
Hypovitaminosis D has reached worrisome proportions in this country. The results of this condition - osteoporosis, fractures, and falls - have become so commonplace, particularly in the elderly, that segments of the general public accept them as natural consequences of aging. Indeed, they have taken their toll in morbidity, direct care expenses, and worsening quality of life. The fact that the initial signs of this deficiency are often silent has made it difficult to identify early, when it can be stopped from progressing to a significant level of morbidity.
This trend can be reversed in the next generation, however, by using such inexpensive measures as a vitamin D supplement, cautiously increased exposure to sunlight, checking of serum vitamin D levels, along with generally good health habits including regular exercise. As simple as this solution may sound, it will be quite challenging to put in place, and will call for us to use our best educational and counseling skills as much as our clinical skills to convince many patients to make changes that will allow them to stay strong, healthy, and independent well into their “old age.”
Practitioners should be more aggressive in screening for vitamin D deficiency in the elderly and in patients with osteoporosis. Ideally, vitamin D deficiency should be corrected before initiation of pharmacologic therapy for osteoporosis.
REFERENCES
- National Institutes for Health Office of Dietary Supplements. Dietary Supplement Fact Sheet: What is Vitamin D? Available at: http://dietary-supplements.info.nih.gov/factsheets/vitamind.asp. Accessed July 23, 2006.
- Holick MF. High prevalence of vitamin D inadequacy and implications for health. Mayo Clin Proc. 2006;81:353-373.
- Bringhurst FR, Demay MB, Krane SM, Kronenberg HM. Bone and mineral metabolism in health and disease. In: Kasper DL, Fauci AS, Longo DL, Braunwald E, Hauser SL, Jameson JL, eds. Harrison’s Principles of Internal Medicine. 16th ed. New York, NY: McGraw-Hill; 2005:2246-2247.
- Wootton AM. Improving the measurement of 25-hydroxyvitamin D. Clin Biochem Rev. 2005;26:33-36.
- Bakhtiyarofva S, Lesnyak O, Kyznesova N, Blankenstein MA, Lips P. Vitamin status among patients with hip fracture and elderly control subjects in Yekaterinburg, Russia. Osteoporos Int. 2006;17:441-446.
- Seeman E, DeIrnas PD. Bone quality: the material and structural basis of bone strength and fragility. N Engl J Med. 2006;354:2250-2261.
- Alberts B, Johnson A, Lewis J, Raff, M, Roberts K, Walter P. Molecular Biology of the Cell. 4th ed. New York, NY: Garland Science; 2002:1305-1306.
- Kaji H, Sugimoto T, Kanatani M, Nasu M, Chihara K. Estrogen blocks parathyroid hormone (PTH)-stimulated osteoclast-like cell formation by selectively affecting PTH-responsive cyclic adenosine monophosphate pathway. Endocrinology. 1996;137:2217-2224.
- Rochira V, Balestrieri A, Madeo B, Zirilli I, Granata ARM, Carani C. Osteoporosis and male age-related hypogonadism: role of sex steroids on bone (patho)physiology. Eur J Endocrinol. 2006;154:175-185.
- Carter GD, Carter R, Jones J, Berry J. How accurate are assays for 25-hydroxyvitamin D? Data from the International Vitamin D External Quality Assessment Scheme. Clin Chem. 2004;50:2195-2197.
- Powell HS, Greenberg D. Takkling vitamin D deficiency. Posgrad Med. 2006 Jun-July;119(1): 25-30
- Holick MF, Siris EF, Binkley N, et al. Prevalence of vitamin D inadequacy among postmenopausal North American women receiving osteoporosis therapy. J Clin Endocrinol Metabol. 2005;90:3215-3224.
- National Osteoporosis Foundation. Physician’s Guide to Prevention and Treatment of Osteoporosis. Washington, DC: National Osteoporosis Foundation; 2003. Available at: www.nof.org/physguide/diagnosis.htm.
- Mauck KF, Clarke BL. Diagnosis, screening, prevention, and treatment of osteoporosis. Mayo Clin Proc. 2006;81:662-672.
- Binkley N. Money for vitamin D is well spent [editorial]. Available at: 222.rheumatologynews.com. Accessed on June 15, 2006.
- Holick MF. Sunlight and vitamin D for bone health and prevention of autoimmune diseases, cancers, and cardiovascular disease. Am J Clin Nutr. 2004; 80:(6 suppl):1678S-1688S.
- Wilkins CH, Birge SJ. Prevention of osteoporotic fractures in the elderly. Am J Med. 2005;118:1190-1195.
- Dawson-Hughes B. Calcium plus vitamin D and the risk of fractures [letter]. N Engl J Med. 2006;354:2286.
- Heaney RP. Nutrition and chronic disease. Mayo Clin Proc. 2006;81:297-299.
- MedlinePlus Herbs and Supplements. Vitamin D. Available at: www.nlm.nih.gov/medlineplus/druginfo/natural/patient-vitamnd.html. Accessed on August 10, 2006.
Table 1. Nutritive Values of Selected Foods: Vitamin D and Calcium
TABLE 1A: VITAMIN D
|
|
|
TABLE 1B: CALCIUM†
|
|
Food Item/Serving Size
|
Vit D, IU
|
|
Food Item/Serving Size
|
Calcium, mg
|
Cod liver oil, 1 tablespoon
|
1360
|
|
Yogurt, nonfat 1 cup
|
490
|
Salmon, cooked, 3.5 oz
|
360
|
|
Soy milk, fortified, 1 cup
|
400
|
Mackerel, cooked, 3.5 oz
|
345
|
|
Orange juice, fortified, 1 cup
|
300
|
Sardines, canned in oil, 1.75 oz
|
250
|
|
Cereal, fortified, 1 cup
|
300
|
Tuna, canned in oil, 3.0 oz
|
200
|
|
Milk, nonfat, 1 cup
|
300
|
Milk, fortified (nonfat, reduced fat, whole)
|
98
|
|
Milk, reduced fat, 1 cup
|
300
|
Margarine, fortified, 1 tablespoon
|
60
|
|
Cheese, Swiss, 1 oz
|
270
|
Pudding, prepared with fortified milk
|
50
|
|
Ice cream, light, ½ cup
|
200
|
Ready-to-eat cereals, fortified, ¾ -1 cup
|
40
|
|
Frozen yogurt, 1 cup
|
200
|
Egg yolk
|
20
|
|
Salmon, canned (with bones), 3.0 oz
|
180
|
Liver, beef, cooked, 3.5 oz
|
15
|
|
Soybeans, cooked, a cup
|
180
|
Cheese, Swiss, 1 oz
|
12
|
|
Cheese, cottage, 1 cup
|
160
|
|
|
|
Pudding, prepared, ½ cup
|
150
|
|
|
|
Almonds, 2 oz
|
150
|
|
|
|
Mustard greens, cooked, ½ cup
|
100
|
|
|
|
Black beans, cooked, 1 cup
|
120
|
Table 2. Clinical Signs Reported for Specific Serum 25(OH)D Levels.
Degree of Deficiency
|
Serum 25(OH)D, nmol/L (ng/mL)
|
Clinical Signs
|
None (normal): Range: >30-50 nmol/L
|
40-50 ng/mL
|
- Lower extremity function and hip BMD increase dramatically up to this level and continue to improve thereafter (although more slowly) up to 100 nmol/L*
|
|
>36-40 ng/mL
|
- Level at which it should remain in the elderly for best muscle strength and balance in lower extremities†
|
|
30-32 ng/mL
|
- Min level for bone health†
|
|
>30 ng/mL (75 nmol/L)
|
- Calcium absorption is maximum*‡; does not improve significantly above this level*
- Minimum steady-state PTH levels seen§
|
Mild/moderate‡
|
<30 ng/mL
|
- Serum PTH levels start to rise
- Asymptomatic¶
|
Chronic, moderately severe§
|
12-30 ng/mL
|
- Secondary hyperparathyroidism
- Metabolic changes: . increased PTH triggers osteoclastogenesis . osteoclasts resorb bone by enzymatic degradation of collagen matrix, HCl . Ca and phosphorus released into extracellular space
- Result: increased bone turnover and skeletal porosity, progressive loss of bone and bone mineral density, increased bone fragility, osteoporosis
|
Chronic, severe§
|
<12 ng/mL (<30
|
|
|
nmol/L) |
- bone pain
- proximal muscle weakness
- Reversible myopathy
|
* Heaney RP. Vitamin D requirements for optimal management of osteoporosis. P. 10.
† Bischoff-Ferrar HI, et al. Am J Clin Nutr. 2004;80:752-758.
‡ Holick MF, et al. J Clin Endocrin Metabol. 2005;90:3215
§ Holick MF. Mayo Clin Proc. 2006;81:353-373.
¶ Bringhurst FR, et al. In: Kasper DL, et al, eds. Harrison’s Principles of Internal Medicine. 16th ed. New York, NY: McGraw-Hill; 2005:2246-2247.
Table 3. Risk Factors for Reduced Bone Mass.
DEMOGRAPHIC
|
CLINICAL
|
BEHAVIORAL
|
Advanced age
|
Family history—osteoporosis
|
Smoking
|
Female sex
|
Family history—hip fracture
|
Low calcium intake
|
Race: white, Asian
|
Lactose intolerance
|
Low vitamin D intake
|
|
Metabolic disorders
|
Low sunlight exposure
|
|
Malignancy (myeloma, lymphoma)
|
Sedentary
|
|
Depression
|
Low body weight
|
|
Hypogonadism (surgical or drug-induced)
|
Excess stress
|
|
Glucocorticoid therapy
|
|
Adapted from: Wilkins CH, Birge SJ. Am J Med. 2005;118:1190-1195. [Table 1, p. 1191]
Table 4. Common Conditions That Can Induce Secondary Osteoporosis.
ENDOCRINE
|
NUTRITIONAL
|
CLINICAL
|
Amyloidosis
|
Anemia, pernicious
|
AIDS/HIV
|
Congenital porphyria
|
Anorexia nervosa
|
Alcoholism
|
Diabetes mellitus, type 1
|
Gastrectomy
|
Ankylosing spondylitis
|
Gaucher’s disease
|
Hypercalciuria
|
COPD
|
Hyperadrenocorticism
|
IBD
|
Leukemia
|
Hyperparathyroidism
|
Inadequate diet (↓Vit D, Ca2+)
|
Liver disease, severe
|
Hyperthyroidism
|
Malabsorption syndromes
|
Lymphoma
|
Hypogonadism
|
Weight loss
|
Mastocytosis
|
|
|
Multiple myeloma
|
PHARMACEUTICAL
|
GENETIC
|
Multiple sclerosis
|
Aluminum
|
Hemochromatosis
|
Organ transplantation
|
Anticonvulsants
|
Hemophilia
|
Rheumatoid arthritis
|
Aromatase inhibitors
|
Hypophosphatasia
|
Scoliosis, idiopathic
|
Cytotoxic agents
|
Thalassemia
|
Spinal cord transsection
|
Glucocorticoids
|
|
Stroke
|
GnRH agonists
|
|
|
Heparin (long-term use)
|
|
|
Immunosuppressants
|
|
|
Lithium
|
|
|
Loop diuretics
|
|
|
Tamoxifen
|
|
|
Thyroxine
|
|
|
TPN
|
|
|
GnRH, gonadotropin-releasing hormone; TPN, total parenteral nutrition; IBD, inflammatory bowel disease; COPD, chronic obstructive pulmonary disease.
Figure 1. Production of active forms of Vitamin D in the liver and kidney.
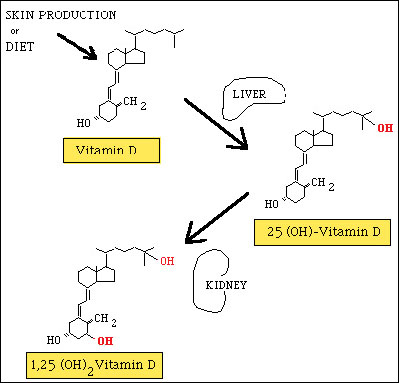
Figure 2. Mechanisms of action of Vitamin D in the body.
Figure is currently not available.
Figure 3. The controversy about “normal” and “ideal” levels of 25(OH)D.
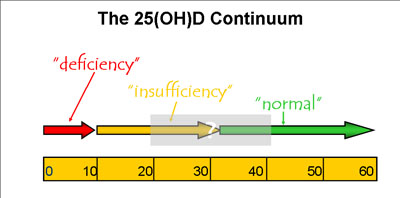
Richard W. Reese, M.D.
Director, Osteoporosis Center of Lancaster
Medical Director, Lancaster Orthopedic Center
Lancaster Rheumatology Associates
2104 Harrisburg Avenue – Suite 24
Lancaster, PA 17604
717-299-1301
rwreese@lha.org