Abstract
Some physicians still give insufficient consideration to the long-term consequences of osteoporosis, even though affected patients who suffer one fragility fracture are three to five times more likely to incur another. Mechanical fixation devices and bone graft substitutes are being used effectively by orthopedic surgeons to restore ambulation for many patients, but the best course of action to treat osteoporosis for the long term, and to maintain patients’ mobility, is a multidisciplinary program involving family internists, orthopedists, and other caregivers.
Background
The statistics are staggering: in the U.S. today osteoporosis is estimated to affect 20 to 30 million individuals. One-third of all women between 60 and 70 years of age, and two-thirds of all women over 80 years of age develop osteoporosis. Their delicate bones carry a 50 percent lifetime risk of a fragility fracture, one-third of which (16 percent) involve the hip. The current annual incidence of fragility fractures approaches 1.7 million (400,000 hip fractures), with an annual healthcare expenditure of approximately $15 billion. These numbers are expected to quadruple by 2050, due, in part, to the aging of our population.1 The number of North Americans more than 55 years old is expected to increase by at least 35 million in the next 20 years.2 Yet, how well this population is able to remain physically active will likely depend, at least in part, upon how well orthopedists diagnose, treat, and prevent age-related disease and injury.
Fragility fractures, defined as those resulting from a low trauma event, affect nearly 50 percent of women and 30 percent of men over the age of 50.3 In the elderly population, osteoporosis is the most common cause of fractures, which occur most frequently in the spine. These fractures occur with significantly increased frequency in female patients 70 years of age or older, and in male patients 80 or older.4 Although fragility (or insufficiency) fractures are ordinarily associated with the elderly and osteoporosis, this is not always the case, as they can occur in any patient with an underlying bone deficiency. (For further discussion of the causes of weakened bone, see Reese: Vitamin D and Bone Disease, in this issue).
The challenge for orthopedic management of these fractures is achieving fixation and stability. Fragility fractures in patients with bone deficiency are particularly common in the spine (vertebral compression), wrist, and hip. While more than 90 percent of fractures occur as the result of a fall, up to 10 percent of hip fractures result from minimal or no trauma, and can occur during routine activities of daily living. The simple stress of standing or sitting down may exceed the compressive strength of bone in the femoral (hip) neck or spinal vertebra. An elderly female with a documented vertebral fracture has a more than 50 percent risk of suffering an additional fracture within one year. Further, once a patient suffers a fragility fracture his or her risk of a future fracture increases 2 to 10-fold.5
History, physical examination, laboratory testing, and x-rays may all be necessary to make the diagnosis and to distinguish simple fragility fractures from other more sinister pathological conditions. Additional diagnostic modalities in less obvious cases include a bone scan (>36 hours), MRI scan, or less commonly, a CT scan. In such cases, in addition to metabolic/endocrine profiling, routine blood work should include a Sedimentation rate, C-reactive protein, and - in males - PSA screening.
Fixation and Stability
Mechanical
Once diagnosed, the orthopedic goals of fracture management are straightforward - reestablish length, alignment, and stability. On occasion, fracture “replacement” may be indicated and necessary to manage pain and to restore function.
In osteoporotic patients with deficient bone, stability is the true challenge. Standard fracture devices (pins, intramedulary rods, plates and screws) often fail, since the bone is inadequate to support them, and develops cavities where the devices are secured. When conventional attempts to reduce or correct the malalignment cannot eliminate the intrinsic instability, specialized orthopedic devices and augmentative implants are needed to overcome the challenge:
In the case of wrist fractures, stability can be improved and length and alignment can be restored by spanning the fracture with external fixation devicesthat are secured with pins and screws peripheral to the injury site. Recently, anatomically configured wristbuttressplates (Figure 1) with locking screws/pins have been developed to enhance stability of the fragments. In these devices, the screws have threaded heads which “lock” into the threaded holes of the plate, thereby not only gaining purchase on the osteoporotic bony fragments, but also maintaining their stable position. Locking plates have also gained popularity in general trauma applications. When combined with a supplemental bone graft at the fracture site, these devices can solve the vast majority of geriatric wrist fractures not amenable to simple closed reduction and casting.
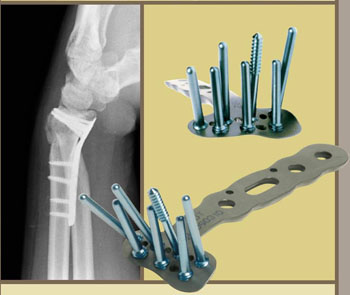 |
Figure 1: “DVR” Locking plate by Hand Innovations; threaded screw holes and drill guides facilitate anatomic positioning. (X-ray shows lateral view of fractured radius.) |
Hip fractures can be divided into three broad categories: subcapital (i.e. in the femoral neck), intertrochanteric, and subtrochanteric (the trochanters are the bony prominences below the femoral neck where the major hip muscles insert). Subcapital fractures are classified by the degree of displacement, but though these anatomical classifications should be useful as a guide to selecting fixation methods, they are not always reliable in patients with advanced osteoporosis. Pinning of even non-displaced or minimally displaced fractures in this population may fail unpredictably and require a second operation to insert a prosthesis, so many surgeons favor preemptive partial hipreplacement (endoprosthesis) for all subcapital fractures (Figure 2). In either case, such a major open procedure in an elderly patient on a semi-emergent basis constitutes a substantial stress that increases the risk of morbidity. Unfortunately, in most cases the surgical options are limited, as many of these fragility fractures occur despite minimal or, in some cases, no trauma. The hip simply fails and the patient falls.
|
Figure 2: Bipolar hip endoprosthesis replaces the fractured femoral
head and is frequently cemented distally. |
Intertrochanteric (and less commonly subtrochanteric) fractures, on the other hand, typically result from a fall and are readily amenable for open reduction internal fixation (ORIF). While the sliding hip compression screw (with or without cement supplementation) has been the gold standard, intramedullary nail devices with an intersecting blade-plate have grown in popularity (Figure 3). They can be done less invasively and provide enhanced biomechanical fixation in selected cases. Additional screws and buttress plates can also be used as necessary.
Bone grafts & substitutes
Wrist and hip fractures present biomechanical challenges that must be addressed with specialized implants and judicious use of bone grafts and/or graft substitutes. As defined, a “bone graft” consists of a portion that contains actual cortical and/or cancellous hard bone (osteoconductive), and a portion that contains bone cells and protein factors that induce the formation of new bone (osteoinductive). Together they can fill the primary defect and stimulate formation of callus at the fracture site. Autogenous grafts from the iliac crest provide both components without the need for allogeneic donor bone, which carries the risks of transmissible agents and a host-versus-graft response.
Donor site morbidity and the expense of local bone banking, however, have spawned the commercial availability of various types of bone graft products, both osteoconductive and inductive. Onlay cortical strut allograft and cancellous “croutons in a bottle” are presently stocked in most busy hospital centers. Combined with the patients’ own blood, the addition of a commercially prepared osteoinductive agent can generate a “super graft.” Such inductive agents include processed de-mineralized bone matrix (bone morphogenic protein-BMD) and/or preparation of other intraoperative blood product (platelet-rich plasma), all of which have been proven to enhance healing and the formation of callus. Osteotrophic factors have been shown to stimulate transformation of marrow progenitor stem cells into osteoblasts, the primary cells in bone matrix formation. These super grafts have been successfully used in open and minimally invasive procedures to enhance healing of fractures in osteoporotic patients. They enhance initial stability and promote healing through early integration into bone.
Other types of bone fillers may likewise provide immediate stability. Polymethylmethacrylate (PMMA) bone cement is an inert two-part polymer used in selected total joint replacements, especially in the elderly. Used judiciously, it has proven most helpful in hip and spinal compression fractures. Performed percutaneously, vertebroplasties likewise have documented benefits in reducing pain and progressive kyphotic deformities for simple fragility fractures with wedge compression. Recently, biocompatible calcium-phosphate compounds. They harden like cement, yet over time are gradually replaced by normal bone (re-substitution), which is typically seen as part of physiologic bony remodeling once primary healing has taken place.
|
Figure 3: Intramedullary devices can provide excellent fixation for unstable peritrochanteric fractures. |
While technical considerations are the initial challenge with any fragility fracture, modulation of the healing process has gained much interest among internists, rheumatologists and orthopedists. Parenteral biophosphonates have been successful in treating osteoporosis through inhibition of normal bone resorption by osteoclasts. They shift the balance of osteoblast/osteoclast activity, and thus increase bone density (as confirmed by DEXA scan and actual bone biopsy). Second and third generation biophosphonates are now available and they not only increase measurable bone density but also decrease the incidence of hip, wrist and spinal compression fractures. Additionally, stimulation of osteoblast proliferation and metabolic activity through hormonal manipulation continues to develop as a viable modality. Calcintonin, mini-dose estrogen, and most recently, recombinant human parathyroid hormone (rhPTH-1,34), have shown great promise when administered through close protocols.
Prevention
The estimated annual cost of fragility fractures in the United States is $15-17 billion.6 Patients who have suffered one fragility fracture are three to five times more likely to have another fracture within one year, especially a spinal compression fracture. Hip fracture patients have a 25 percent higher risk of mortality within one year; a 25 percent risk of losing their ability to earn a living and their independence (likely leading to nursing home placement); and a 50 percent chance of never regaining pre-fracture ambulation. For these reasons, the need to treat these patients aggressively and initiate post-discharge medical protocols for untreated osteoporosis has never been stronger.
To improve awareness of fragility fractures in the osteoporotic patient, it is necessary to maintain ongoing education of referring physicians, medical staff, and patients. One research study conducted by St. Michael’s Hospital in Toronto, Canada, found that a coordinated post-fracture osteoporosis education and treatment program for patients with fragility fractures and their caregivers resulted in more than 95 percent of the patients being diagnosed, treated, and referred for osteoporotic care.7 Western Infirmary Hospital and other leading centers in Glasgow, Scotland have championed these measures and made a substantial positive impact on morbidity and national healthcare costs.8 Through multidisciplinary efforts currently underway at Lancaster General Hospital, similar protocols have been set in motion to ensure that patients with fragility fractures are evaluated and educated, and that appropriate management is initiated with follow-up.
The essential steps to ongoing care in an osteoporotic patient who suffers a fragility fracture include:9
- The patient must be identified as an individual with a high risk for future fracture.
- Responsibility for ongoing evaluation and treatment must be assumed by a specific physician or caregiver.
- Clinical testing must be performed to diagnose osteoporosis.
- Etiology of the patient’s low bone density should be determined.
- Osteoporosis should be treated pharmacologically or with other means.
- Patients should be educated about home safety, fall prevention, and protection of their injured site from further damage.
- Patients should receive routine follow-up evaluations.
Conclusions
New and better fixation devices as well as bone graft substitutes are providing additional surgical options for the osteoporotic patient who suffers a fragility fracture. These advances increase the likelihood that patients can maintain their mobility. Yet, as surgeons who care for these patients, our goal should be more than stability and fixation of the fracture. We work as part of an orthopedic/medical team to definitively determine the etiology of the fracture, fix the fractured bone, and then develop ongoing treatment and evaluation plans specific to the osteoporotic patient. We maintain a keen awareness and education about osteoporosis and its life-long impact on patients, and work in tandem with primary care physicians and other clinicians to ensure these patients receive the education and long-term care they need to prevent future fractures, and to enhance their quality of life.
- Orwoll, Eric S., Bliziotes, Michael. Osteoporosis: Pathophysiology and Clinical Management. NEJM. 348:2269-2270, 2003.
- Statistical Information Staff, Population Division, U.S. Census Bureau. Projections of the total resident population by 5-year age groups, and sex with special age categories: middle series, 2016-2020. Washington, DC: U.S. Census Bureau; www.census.gov/population/projections/nation/summary/np-t3-e.txt
- Bouxsein, Mary L., Kaufman, John, Tosi, Laura, Cummings, Steven, Lane, Joseph, Johnell, Olof. Recommendations for Optimal Care of the Fragility Fracture Patient to Reduce the Risk of Future Fracture. J Am Acad Orthop Surg. 12(6):385 395.
- Rodan, Gideon, Reszka, Alfred. Osteoporosis and Bisphosphonates. J Bone Joint Surg AM. 85:8-12, 2003.
- Bogoch, Earl R., Elliot-Gibson, Victoria, Beaton, Dorcas E., Jamal, Sophie A., Josse, Robert G., Murray, Timothy M. Effective Initiation of Osteoporosis Diagnosis and Treatment for Patients with a Fragility Fracture in an Orthopaedic Environment. J Bone Joint Surg Am. 88: 25-33, 2006.
- 6. Hoerger, TJ, Downs, KE, Lakshamanan, MC, Lindrooth, RC, Plouffr I, Wendling, B, West, SL, Ohsfeldt, RL. Healthcare use among US women age 45 an older: total costs for selected postmenopausal health risks. J. Women’s Health Gender Based Medicine. 8:1077-89, 1999.
- Bogoch, Earl R., Elliot-Gibson, Victoria, Beaton, Dorcas E., Jamal, Sophie A., Josse, Robert G., Murray, Timothy M. Effective Initiation of Osteoporosis Diagnosis and Treatment for Patients with a Fragility Fracture in an Orthopaedic Environment. J Bone Joint Surg Am. 88: 25-33, 2006.
- Ray, NF, Chan, JK, Thamer, M, Melton, LJ. Medical expenditures for treatment of osteoporotic fractures in the US in 1995: report for the National Osteoporosis Foundation. J. Bone Miner Res. 12:24-35, 1997.
- Kaufman, John D., Bolander, Mark E., Bunta, Andrew D., Edwards, Beatrice J., Fitzpatrick, Lorraine, Simonelli, Christine. Barriers and Solutions to Osteoporosis Care in Patients with a Hip Fracture. J Bone Joint Surg. Am. (85): 1837-1843. 2003.
J. Paul Lyet, M.D., Ph.D.
Medical Director, Lancaster General Orthopedic Center
Lancaster Orthopedic Group
231 Granite Run Drive
Lancaster, PA 17601
717-560-4200
jplyet@lancasterortho.com
|